Active defects show self-constraint in spontaneous flows
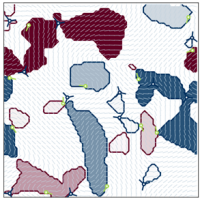
Study suggests how biological fluids balance motion while protecting structure.
Biology is continually driven by motor proteins and other active machinery that gives biomaterials such distinct properties. This intrinsic activity keeps living systems away from equilibrium (i.e. not dead), generates structure, and powers movement. It is known that spontaneous, collective motility naturally arises in active fluids. However, these collective flows tend to exhibit a form of disorderly ‘active turbulence’ which chaotically mixes biological materials. On the other hand, biological functionality requires that self-organised structures are protected — we wouldn’t want our internal organs to be mangled whenever we go for a stroll! So how is it that active flows can be disorderly while simultaneously preserving self-organised structure?
To investigate this, researchers from the School’s Institute for Condensed Matter and Complex Systems, along with collaborators from ESPCI Paris-Tech in France, focussed on an experimentally realisable active film composed of biological filaments (microtubules) and motor proteins (kinesin). When the motor proteins burn biochemical fuel, they actively push the filaments to orient and flow.
While most of the filaments align, the orientation field is riddled with points where the orientation is suddenly singular. These singular points are called topological defects and activity causes pairs of defects to be continually created and annihilated—similar to electron/positron pair creation/annihilation. Active systems are different in that the activity causes the positive defects to be self-motile, zipping around the system until they annihilate.
It was previously noted that self-motile defects tend to be found at the edge of vortices. However, we compared the vorticity and strain rate at each point in the film and discovered that defects are not simply found near vortex edges; rather, they are tightly constrained to be found exactly on lines where vorticity and strain rate perfectly balance. The team were able to explain this spontaneous self-constraint between defects and mesoscale coherent flow structures through the existence of ‘bend walls’ — elongated narrow kinks in the orientation, akin to Néel walls in magnets. Positive defects move along the bend walls, which themselves actively drive flows with balanced vorticity and strain rate.
The findings indicate that defects are not simply points, despite being topological singularities. Rather they are just one part of larger structures involving defects, bend walls and coherent flows. The self-constraint discovered suggests how biological systems may balance motion while protecting functional structures.
Dr Tyler Shendruk, School of Physics and Astronomy commented:
This was an exciting and fast-moving project. It started as an exploration of different ways to quantify flow structures that rapidly led to surprising results. Fortunately, we quickly established an international collaboration that involved wonderful experimentalists and other computational physicists. The collaboration tested our predictions and established just how general our conclusions are.
PhD student Louise Head, School of Physics and Astronomy said:
We are excited to see the future work that can arise from our discovery that the dynamics of topological defects are interwoven with bend walls and lines of simple shear.