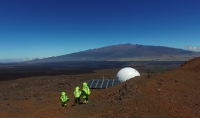
University of Edinburgh and UK Centre for Astrobiology PhD student Samuel Payler completes NASA’s 8-month long HI-SEAS Mars isolation mission.
HI-SEAS (Hawai’i Space Exploration Analog and Simulation) is a habitat on an isolated Mars-like site on the Mauna Loa side of the saddle area on the Big Island of Hawai'i. HI-SEAS studies the impacts of long term isolation on crew performance and cohesion in preparation for future missions to the red planet. Samuel was serving as the Science Officer on the mission.
Prior to this project, Samuel worked on the UK Centre for Astrobiology’s MINAR program, and NASA’s BASALT project, both of which gave him the opportunity to learn about and contribute to aspects of technology development and mission support architectures for future Mars exploration. HI-SEAS has now provided him with the astronaut’s side of space exploration.
Samuel commented: “Knowing that information acquired from our mission will contribute to overcoming the problems caused by isolation, is well worth all the personal challenges imposed by living in a dome on the side of an active volcano with only shelf stable foods and five other people to talk to. Luckily participation was made much easier by having an excellent crew. Everyone worked extremely hard on everything from task work to personal relationships. We started the mission as strangers and are leaving as close friends."
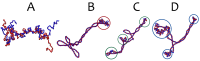
Topology plays a key role in biophysics, from genome organisation to cellular motion. Our work suggests a new role in DNA melting.
When a double-stranded DNA helix is heated up, the H-bonds keeping the double helix together break, and DNA melts into two single strands. Long ago, experiments revealed that DNA melting in circular DNA is much smoother than in linear DNA. Why is this the case? Most theories attempted to identify a mechanism turning the abrupt, first-order transition of linear molecules into a smooth, second-order one. Our simulations led us to an alternative explanation, based on the fact that circular DNA needs to conserve the linking number between its two strands.
Instead of a traditional transition between zipped and melted state, increasing the temperature takes the system into a coexistence region between a denaturation bubble of unlinked single-stranded DNA, and a double-stranded phase where the DNA linking lost in the bubble is stored as writhe (so the double-stranded section looks like a coiled up phone cord). This coexistence region can extend over a wide temperature range, with the DNA fraction in the bubble and double-stranded phases varying gradually with temperature, which may account for the experimental results. Our results suggest new single molecule experiments to be performed on topologically-constrained DNA melting.
Dr Davide Michieletto reported "Topology plays a key role in biophysics but its understanding is often limited by the lack of a suitable theoretical framework. In this work we have managed to put together theory and simulations to give a qualitative description of how topology affects DNA melting that explains existing experimental observations."
Students Enrique Cervero and Hamish Geddes who form part of the University of Edinburgh Hyperloop Team, known as HypED, worked for over ten months to design and build a Hyperloop prototype, a method of levitating transportation propelled along a vacuum tube.
Much of their summer was spent at the mechanical lab in King’s Building drawing sketches, tightening bolts and drilling holes with the ultimate purpose of bringing their Hyperloop Pod, Poddy McPodface, to life.
All this work culminated at the end of August in Los Angeles, where HypED was invited by SpaceX to participate in the finals of one of the most prestigious engineering competitions in the world: The Hyperloop Pod Competition II. A total of 25 teams from all over the world were invited to unveil and race their prototypes at the space company’s headquarters, HypED being the only British team and one of four European teams.
The team arrived in LA about a week before the competition where they brought their prototype to a local workshop in Los Angeles, Urban Workshop, where they spent most of the pre-competition days giving the final touches to the design.
Enrique Cervero: "Our main worry before the competition was that we would not finish our pod in time, that there would be some flaw or eventuality that we had not planned for and that would ultimately prevent us from competing at SpaceX. We therefore worked hard trying to get everything done perfectly to meet SpaceX’s requirements."
When the pod was complete, they drove it to SpaceX in Hawthorne, LA, where it was tested for safety, systems and functionality before the competition. Out of the 25 teams who got invited to the competition, only 3 would be allowed to test their pod and race it in the vacuum tube.
HypED’s prototype was unfortunately not one of the 3 chosen by SpaceX. However, the team was given clearance to test their pod at a speed of 40m/s (144km/h) in the vacuum track, which would have made it one of the fastest Hyperloop Pods ever tested.
Enrique Cervero commented: "Over the entire year and competition, I have learned that real world applications of engineering are never simple and require a level head and persistence to complete: there must be a lot of thought put into a design, many drafts, scraps and failures need to be done before arriving at the finalised product. I have also acquired a lot of technical experience, how to use industrial machinery, solve real world mechanical problems and work as a team to bring our ideas to life."
The outcome of the competition was also an imperative learning experience for the team which they will use to their advantage in next year’s competition. They will learn from their design flaws and mistakes and remove them in their next design, use the advice and knowledge given to them by Tesla and SpaceX engineers, and improve on the design’s advantages.
Image gallery
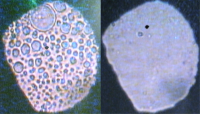
Oil and water don’t mix. This is why a bottle of French dressing has to be shaken before use and why, when an oil tanker sinks, the oil that is spilled can be scooped off the surface of the sea.
The tendency of oil molecules to avoid mixing with water (called hydrophobicity) is very common and has many effects and applications. Soap and detergent contain molecules that have an oily (hydrophobic) 'tail' and a water-loving (hydrophilic) 'head' so that when a greasy plate is washed the oily tails bind to the grease and the heads bind to the water, forming microscopic blobs of grease that are happy to disperse in the water. The membrane enclosing cells in all living things are formed from similar molecules, which organise themselves to form sheets so that they can bury their water-hating tails inside the membrane, exposing their water-loving heads to water on the outside.
Now a group of physicists in the Centre for Science at Extreme Conditions at the University of Edinburgh have shown in a paper to be published in Science Advances that there are circumstances where a water-hating molecule can be made to mix with water. They studied water and methane (the simplest possible oil and the major component of natural gas) at pressures up to and beyond 20,000 times atmospheric pressure (20 times higher than the pressure at the bottom of the ocean: Marianas Trench). A mixture of methane and water 0.1mm in diameter and 0.05mm thick was squeezed between two diamonds and the behaviour was observed under a microscope. At low pressures methane and water don’t mix and, as the left hand picture shows, droplets of methane become surrounded by water. As the pressure is increased above 13,000 atmospheres, the methane droplets start to shrink and eventually disappear, so that the sample becomes a uniform liquid mixture of methane and water as shown in the right hand picture. The Edinburgh team were able to estimate the amount of mixing from the relative areas of the droplets in pictures like the one on the left. They found that the amount of mixing increased from less than 1% at 13,000 atmospheres to almost 50% at 20,000 atmospheres, so that 4 of every 10 molecules in the mixture is a methane molecule.
Discovering conditions under which oil and water do mix has far-reaching implications. Methane and water occur widely throughout the solar system: within the Earth, in Neptune and Uranus, and in Titan and Triton. The new finding from Edinburgh will therefore enable scientists to build more accurate models of these planets and satellites. The finding also offers possibilities for the green agenda: it holds out the prospect of extending considerably the range of what is surely the greenest of all solvents: water. To make this practicable however, further research is necessary to reduce the pressure at which oil-water mixing can occur. In the meantime, the discovery has given scientists a simple system in which the love-hate relation between water and oily molecules - which is important for everything from washing up to life itself - can be 'tuned' at will and so probed and understood in new ways.
Scientists have helped solve the mystery of what lies beneath the surface of Neptune, the most distant planet in our solar system.
Extremely low temperatures on planets like Neptune – called ice giants – mean that chemicals on these distant worlds exist in a frozen state. Frozen mixtures of water, ammonia and methane make up a thick layer between the planets’ atmosphere and core – known as the mantle. However, the form in which these chemicals are stored is poorly understood.
Using laboratory experiments to study these conditions is difficult, as it is very hard to recreate the extreme pressures and temperatures found on ice giants. Instead, scientists at Edinburgh ran large-scale computer simulations of conditions in the mantle. By looking at how the chemicals there react with each other at very high pressures and low temperatures, they were able to predict which compounds are formed in the mantle.
"Computer models are a great tool to study these extreme places, and we are now building on this study to get an even more complete picture of what goes on there." Dr Andreas Hermann, Centre for Science at Extreme Conditions
The team found that frozen mixtures of water and ammonia inside Neptune – and other ice giants, including Uranus – likely form a little-studied compound called ammonia hemihydrate. The findings will influence how ice giants are studied in future and could help astronomers classify newly discovered planets as they look deeper into space.
The study, published in the journal Proceedings of the National Academy of Sciences, was supported by the Engineering and Physical Sciences Research Council.
The work was carried out in collaboration with scientists at Jilin University, China.
Dr Andreas Hermann also reported: "This study helps us better predict what is inside icy planets like Neptune. Our findings suggest that ammonia hemihydrate could be an important component of the mantle in ice giants, and will help improve our understanding of these frozen worlds."
A study of distant galaxies has enabled astronomers to make the most accurate measurement to date of the large-scale structure of the universe.
Their findings support a theory that about 96 per cent of the Universe is made of elusive dark energy and dark matter. In making their discovery, the international team including researchers at the University of Edinburgh, created the largest map so far of dark matter in the cosmos. Their results draw on data collected during the first year of an international project called the Dark Energy Survey (DES).
Scientists taking part seek to better understand dark energy, which may be responsible for the accelerating expansion of the universe. Their latest measurements of the amount and distribution of dark matter in the present-day cosmos are as precise as information captured previously about the early universe, by the European Space Agency’s orbiting Planck observatory. Having both sets of data enables scientists to understand more about how the structure of the universe has evolved over 14 billion years.
For the latest study, researchers used data from a powerful dark energy camera on a telescope in Chile to create maps of galaxy positions as tracers and trace the density of dark matter. They also precisely measured the shapes of 26 million galaxies, and used a technique called gravitational lensing – which accounts for light bending over large distances – to directly map the patterns of dark matter’s gravity over billions of light years. To make these ultra-precise measurements, the DES team developed techniques to detect the tiny lensing distortions of galaxy images – which are invisible to the eye – enabling advances in understanding these signals.
The DES is a collaboration of more than 400 scientists from 26 institutions in seven countries. Scientists at the participating Universities of Edinburgh, Cambridge, Manchester, Nottingham, Sussex, Portsmouth and UCL have led work central to the team’s results.
Their primary instrument, the 570-megapixel Dark Energy Camera, is mounted on the 4-metre Blanco telescope at the Cerro Tololo Inter-American Observatory in Chile.
UK researchers have led, over the years, major science analyses central to the results presented today. DES scientists are using the camera to map one-eighth of the sky in unprecedented detail over five years.
The study was supported by the Science and Technology Facilities Council.
Dr Joe Zuntz of the University of Edinburgh reported: “The DES measurements, when compared with the Planck map, support the simplest version of the dark matter and dark energy theory. This is a huge part of the puzzle of how the cosmos has evolved over the past 14 billion years. It’s the dark Universe made visible on an unprecedented scale.
Image gallery
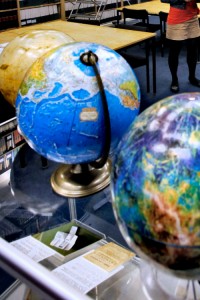
Students Estifa’a, Leevan and Lorenzo will soon be undertaking their research project at a partner institution overseas. These students, who have completed year 3 of their undergraduate degree, are the first of our students to embark on their research project overseas as part of the MPhys Physics with a Year Abroad degree.
Estifa’a will be working with scientists from the Canadian group, ALPHA – Canada, a group in the international ALPHA project based at CERN. This group studies the properties of antimatter atoms in order to attempt to answer some of the most fundamental questions in modern physics. Having previously successfully isolated antimatter atoms in a “magnetic bottle”, their research now focuses on studying the gravitational behaviours of these atoms, as well as using microwave spectroscopy to study the charge, parity and time reversal of anti–hydrogen atoms.
I wanted to embark on a journey that will push me outside of my comfort zone, challenge my perceptions and help me gain self-confidence. I will have the chance to meet people from diverse backgrounds in a research environment outside the UK. It was also the idea of being involved in physics research that has a real effect on our understanding of science that really excited me, as well being a part of a research team that is trying to make scientific contributions of historic importance. Estifa'a
Leevan’s work is a multi-disciplinary project with collaborations between Neuroscience, Chemistry and the Bio21 institute in Melbourne. It will focus on the use of fluorescent nanodiamonds for biological imaging.
This year abroad opportunity will provide me with the perfect platform from where I will be able to both experience what the field of medical physics research would entail and what a life would be like after university if I continued my academic work and became a researcher. Leevan
Lorenzo will be taking part in a research project at TRIUMF, which is one of the world’s leading subatomic physics laboratories, based in Canada, focusing on particle, nuclear and accelerator physics. He will be joining the DRAGON experiment, which aims at recreating and studying nucleosynthesis processes that happen within the cores of stars as a product of high energy nuclear fusion reactions.
This placement will give me the opportunity to have first-hand experience of what a career in Experimental Physics would be like, therefore potentially helping me in further career choices. Lorenzo
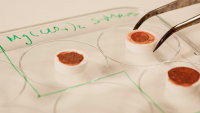
Scientists have discovered that UV light can ‘activate’ perchlorate under Martian environmental conditions, causing it to enhance the rate of bacterial death.
Professor Charles Cockell and Jennifer Wadsworth have discovered that perchlorate, which is a molecule that is abundant on the surface of Mars, can enhance the rate of bacterial death when 'activated'. Perchlorate is usually very stable at room temperature/colder temperatures and you have to heat it to hundreds of degrees Celsius to activate it. However, it has been shown that UV light can 'activate' it under Martian environmental conditions. They suspect the UV light causes it to decay into a different molecule which is lethal for bacteria.
The other major finding is that if you mix other components of the Martian surface (iron oxides and hydrogen peroxide) with the perchlorate, the lethal effect on bacteria is increased. This is interesting because it is generally thought that it would not be possible to activate perchlorate under Martian conditions. Astrobiologists will take this into consideration when looking for biomarkers/life on Mars.
Jennifer Wadsworth commented: "We already know the surface is probably inhospitable, so future missions might look at potential subsurface environments for life. We don’t know exactly how far the effect of UV and perchlorate would penetrate the surface layers, as the precise mechanism isn’t understood. If it’s the case of altered forms of perchlorate diffusing through the environment, that might extend the uninhabitable zone. We may have to dig a little deeper to find a potential habitable environment."
Simulations and theory indicate that the “synchronized swimming” of bacteria occurs in much sparser suspensions of the microorganisms than expected.
While being one of the most important unsolved questions in physics, the phenomenon of turbulence is not commonly associated with microorganisms.
Yet experiments on suspensions of swimming bacteria have revealed that they exhibit chaotic collective motion reminiscent of the turbulence seen around an airplane wing or in a waterfall: when the concentration of bacteria is high enough, they start to swirl around in vortex-like patterns that constantly change size and direction. The mechanism of this phenomenon is thought to be related to the mutual reorientation of bacteria in the fluid flow they create while swimming, but the precise mechanism has proven to be elusive.
In a recent paper, researchers from Lund, Cambridge and Edinburgh add another piece to this puzzle. Using theory and large-scale computer simulations, Joakim Stenhammar, Cesare Nardini, Rupert Nash, Davide Marenduzzo, and Alexander Morozov demonstrate that even at very low densities, individual microorganisms "feel" the presence of the others and their motion is thus never fully independent. As the concentration of bacteria is increased, the strength of these correlations build up to eventually form a chaotic state strongly similar to that seen in experiments.
Dr Alexander Morozov commented: "Up to now it was thought that low-density suspensions of swimming microorganisms are random and featureless, and that the correlations appear suddenly at the onset of collective motion. Surprisingly, we see measurable signs of these correlations at very low densities of microswimmers that significantly affect physical properties of the suspension."
Image gallery
Astronomers have released the largest ever map of the sky at infrared wavelengths, with over 1.5 million mega-pixels and containing nearly two billion stars and galaxies.
The largest infrared image of the sky ever taken, known as the UKIRT Hemisphere Survey, has been released today by astronomers led by Dr Simon Dye at the University of Nottingham and Professor Andy Lawrence at the University of Edinburgh.
The image is a whopping 1.5 million mega pixels and has detected nearly two billion stars and galaxies. It is the culmination of over ten years work on an international project to image the northern sky in the infrared part of the spectrum. Astronomers can search the image using an online database based in Edinburgh. Initially the survey is open to UK astronomers along with colleagues in Hawaii and Arizona, but in a year’s time it will be open to all astronomers world wide.
The image has been captured using the United Kingdom Infrared Telescope (UKIRT). This is a 3.8m telescope located near the summit of Mauna Kea, a dormant volcano on Hawaii. The telescope is dedicated to observing the sky in the infrared part of the spectrum, giving astronomers a view of the processes which shape the formation of stars and galaxies at wavelengths invisible to human eyes. The telescope uses an innovative IR Wide Field Camera developed at the Astronomy Technology Centre of the Royal Observatory, Edinburgh.
Dr Dye commented: “Releasing this image is a hugely exciting moment for astronomy. It gives the clearest view we have ever seen of the northern sky in the infrared. This is a significant milestone, providing an important database with a high legacy value for astronomers in years to come.”
Professor Andy Lawrence reflected: “The survey has already been used to find some of the most distant quasars known. I am cooking up my own projects as we speak.”
Completion of the project has been made possible by a partnership between the UK Science Technology and Facilities Council, the University of Hawaii, the University of Arizona, Lockheed Martin and NASA.