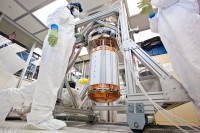
The Large Underground Xenon (LUX) dark matter experiment, which operates beneath a mile of rock at the Sanford Underground Research Facility in the Black Hills of South Dakota, has completed its search for the missing matter of the universe – one of the most sought for goals in physics and astronomy. Final results are published in Physical Review Letters, with an editorial comment to highlight their significance.
Since 2013, the experiment has led worldwide direct searches for dark matter, in which evidence for the scattering of dark matter particles in a terrestrial detector is sought. The final result from the 2014-2016 run, recently accepted for publication in PRL, pushes the sensitivity of the instrument to a final performance level that is 4 times better than originally expected, and a further improvement on its existing world lead. Despite seeing no sign of a signal, the results rule out large fractions of the theoretical parameter space, constraining tightly the nature of dark matter.
Data analysis continues, searching for other possible rare signals in the data. The instrument itself is now being decommissioned and removed from the deep underground laboratory, in preparation for installation of its successor, LUX-ZEPLIN. Expected to begin taking data in 2019, this instrument is over twenty times larger, and expected to be over a hundred times more sensitive still. The University of Edinburgh is one of the leading institutions in LUX, contributing the Executive Chair of the collaboration, and is a participant in LZ.
These results reflect that LUX is now some twenty times more sensitive to dark matter than any of its predecessors. While if Nature had been kinder, we might have made a discovery, our results have greatly narrowed the possibilities for what dark matter might be - itself an important result. A new generation of experiments are now coming online or being built, including our own LUX-ZEPLIN. The search for dark matter remains a top priority. Says Prof Alex Murphy, The University of Edinburgh
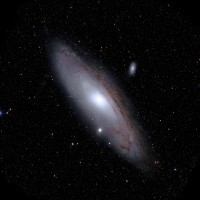
Astronomers around the world are poised to discover billions of new stars, galaxies and solar system objects, thanks to newly released data from a major survey of the skies involving University of Edinburgh researchers.
Scientists are poised to make fresh discoveries about the Universe, thanks to newly released data from a major astronomy survey. Results from a four-year project to capture digital images of the sky should enable astronomers around the world to discover billions of new stars, galaxies and solar system objects. The project, involving Edinburgh scientists, will also enable researchers to study the farthest reaches of the universe and gain insights into elusive dark energy and dark matter.
Images in the study have been taken using a 1.8-metre telescope at the summit of Haleakalā on Maui, Hawaii. The technology is known as the Panoramic Survey Telescope & Rapid Response System (Pan-STARRS). It captured images of patches of sky - each about 36 times the area of the moon - every 30 seconds for four years. The telescope’s regular imaging means that fast moving objects and exploding stars can be tracked across nearly the whole sky. Images from the project, being released by the Space Telescope Science Institute and the University of Hawaii, can be analysed to identify and catalogue astronomical objects. Data from the survey has been shared in advance with astronomers in the team. Already, this has led to discovery of hundreds of supernovae – exploding stars that give off massive amounts of energy as they die. It has enabled scientists to see individual stars in nearby galaxies, and to discover giant streams of stars in our own Milky Way. The survey dataset is being released in two stages. The first gives an average value for the position, brightness, and colours of all the recorded objects in the sky at a particular point in time.
A further release next year will provide a catalogue of information, and allow access to individual images for each time frame. The database will include information on individual snapshots of a given region of sky.
The Pan-STARRS project was undertaken by an international collaboration including the Universities of Edinburgh and Durham, and Queen’s University Belfast.It was supported by NASA and the National Science Foundation.
“This rapid, repeating survey has enabled us to discover very rare events in which a massive black hole shreds a passing star, which otherwise would have been impossible to spot. Releasing the data will now enable astronomers round the world to study huge numbers of distant stars and galaxies in ways we can't even guess." said Professor Andy Lawrence, of the University’s School of Physics and Astronomy
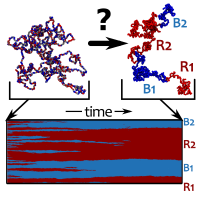
A new theoretical model of DNA as a "recolourable" polymer may explain why specific "epigenetic" patterns on the genome can survive from one cell generation to the next and allow cellular memory.
Every cell in our body contains the same DNA. Nevertheless, a brain cell is clearly very different from, say, a liver cell, in terms of which genes are active and inactive. The specialisation into different types of cells is made possible by "epigenetics" (literally, "beyond the genetic information"). Epigenetics acts through biochemical markers called "post-translational modifications" which are deposited onto histone proteins -- the proteins needed to package the DNA inside the nucleus. Epigenetic marks vary from cell to cell, allowing each cell to have its own identity. Nonetheless, epigenetic marks are very dynamic: they are gained and lost by histones all the time, and many are removed during replication. This then begs the questions, how does a cell remember its own identity throughout its life and how is its identity passed down to the progeny cells when it divides?
Davide Michieletto, in collaboration with Davide Marenduzzo and Enzo Orlandini (Padova) have formulated a new model to address these questions. The model treats the complex of DNA and histones (also called "chromatin") as a polymer made of beads, where each bead corresponding to a few histones (with their corresponding DNA). In the model, the polymer beads are "coloured" based on their epigenetic state, and this can be changed dynamically mimicking the action of "writer" proteins, enzymes found in the cell. The epigenetic colour is then recognised by "readers", which model chromatin-bridging proteins which have an affinity for a given epigenetic mark. The model displays an abrupt first-order-like transition between a swollen and epigenetically "disordered" chromatin and a collapsed and "ordered" one, where the whole polymer has the same colour. The first order nature of the transition is important: it implies that there is hysteresis and memory, and the researchers have shown that, following a (simulated) replication event, the system is able to return to the same ordered state (so the corresponding cell will keep its identity).
The findings therefore strongly suggest that epigenetic memory may be made possible through a positive feedback loop between "reader" and "writer" proteins which couple the 1D epigenetic patterning to the 3D chromatin folding.
Dark matter, the elusive material that accounts for much of the Universe, is less dense and more smoothly distributed throughout space than previously thought, according to a new study co-led by astronomers at the University of Edinburgh. The international team of scientists studied wide-area images of the distant universe, taken from the European Southern Observatory in Chile. They applied a technique based on the bending of light by gravity - known as weak gravitational lensing - to map out the distribution of dark matter in the Universe today. Their study represents the largest area of the sky to be mapped using this technique to date.
The new results contradict previous predictions from a survey of the far-off universe, representing a point in time soon after the Big Bang, imaged by the European Space Agency's Planck satellite. This previous study used a theoretical model to project how the Universe should appear today. The disagreement between this prediction and the latest direct measurements suggests that scientists' understanding of the evolving modern day Universe is incomplete and needs more research.
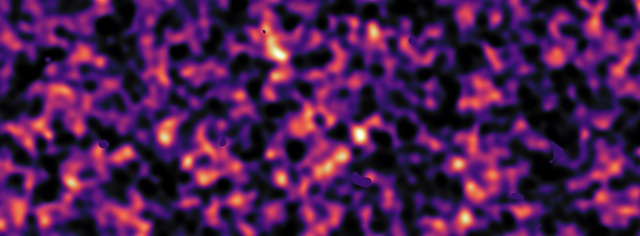
The latest study, published in Monthly Notices of the Royal Astronomical Society, was carried out by a team jointly led by the University of Edinburgh, the Argelander Institute for Astronomy in Germany, Leiden University in the Netherlands and Swinburne University of Technology, Australia in an ongoing project called the Kilo Degree Survey, or KiDS. It was supported by the European Research Council.
"Our findings will help to refine our theoretical model for how the Universe has grown since its inception, improving our understanding of the modern day Universe.” says Dr Hendrik Hildebrandt of the Argelander Institute for Astronomy in Germany.
"This latest result indicates that the cosmic web dark matter, which accounts for about one-quarter of the Universe, is less clumpy than we previously believed.” said Dr Massimo Viola of Leiden University in the Netherlands.
"Unravelling what has happened since the Big Bang is a complex challenge, but by continuing to study the distant skies, we can build a picture of how our modern Universe has evolved.” said Professor Catherine Heymans of the University of Edinburgh's School of Physics and Astronomy.
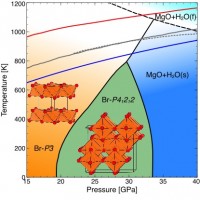
Water inside Earth’s interior is crucial to assist mantle convection. Without it, mantle convection would be inefficient, volcanic activity would cease, as would plate tectonics on the surface.
Hydrous minerals help transport water deep into Earth’s mantle, and form part of a cycle that regulates the sustained presence of water, both in the interior and on the surface, on geological time scales. To understand the deep-water cycle, it is crucial to study the properties of hydrous minerals under the conditions present in Earth’s mantle. Brucite, chemical formula Mg(OH)2, is one of the simplest hydrous minerals and stores significant amounts of water in the form of hydroxyl groups. It is assumed to decompose in the mantle transition zone, but recent work by Dr Andreas Hermann, with collaborators from Florida State University, shows that the mineral can exist in a more compact high-pressure phase instead, which pushes the stability region of brucite several hundreds of kilometers deeper and into the lower mantle. Brucite might be present at much greater depths, and in much larger quantities, than previously thought. This changes the current thinking about the water storage capacity of the deep Earth, and the role hydrous minerals play in transporting and holding water inside our planet.
The work involved quantum-mechanical calculations that screened thousands of potential crystal structures of brucite under extreme pressure conditions. The new phase, which has a network structure akin to anatase, emerged eventually from those calculations. Besides being more stable than the known brucite structure at high pressures and temperatures, Dr Hermann and his collaborator showed that the new phase has a unique elastic response, which might enable its direct detection in seismic signatures, as well as specific vibrational properties that should make its detection in laboratory experiments straightforward.
The research is published in the Proceedings of the National Academy of Sciences.
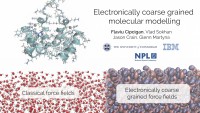
Flaviu, who is a final year CASE student with NPL has won the thesis presentation award at the first NPL Post Graduate Institute for his presentation titled 'Electronically Coarse Grained Molecular Modelling'.
Congratulations to Flaviu for winning the best presentation award at the first annual Postgraduate Institute conference at NPL. The conference was attended by 150 delegates over two days, comprising postgraduate researchers based both at NPL and partner universities, their supervisors, invited guests and speakers.
A number of the postgraduates also took part in a challenge to describe their doctoral work comprehensively in just three minutes, with just one slide. The winner was Flaviu Cipcigan, for his presentation 'Electronically Coarse Grained Molecular Modelling',
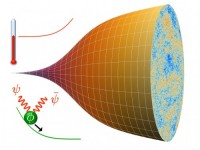
Warm inflation is an alternative and simpler dynamical picture for the the early Universe, in which the dominant expansion period occurs in a warm phase, whereas in the standard inflation picture, accepted for over three decades, it occurs in a cold phase. This leads to distinct differences in the history of the early Universe and present-day observational signatures.
For over the two decades since warm inflation was suggested by Arjun Berera, experts in the fields of cosmology and particle physics have regarded it as almost impossible to realize this idea in a simple first principles model. This paper has confounded these expectations and developed a simple and compelling first principles model of warm inflation. This now provides a solid theoretical foundation to an alternative paradigm for the early Universe, which shows excellent agreement with large scale observational data (see figure below).
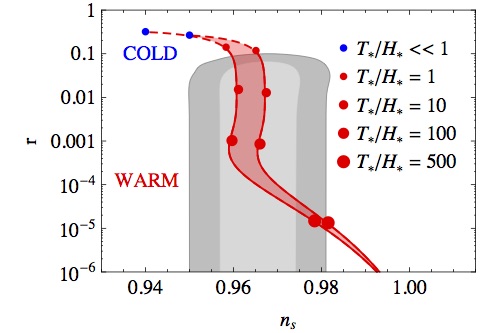
In standard inflation, any preexisting radiation is stretched and dispersed during a brief cosmic phase and no new radiation is produced. The Universe’s temperature plummets by several orders of magnitude, and an ensuing reheating period fills the Universe with radiation again. Warm inflation is simpler. New radiation is constantly produced by the decay of the scalar field that triggers inflation, the inflaton; the temperature remains large; and there is no reheating phase. Ironically, however, models of warm inflation have so far required thousands of additional fields to be coupled to the inflaton to avoid large corrections to its mass.
This paper treats the field driving inflation as a pseudo Nambu-Goldstone boson of a broken gauge symmetry and its mass is naturally protected against large quantum and thermal corrections. The model is similar to the Little Higgs mechanism for electroweak symmetry breaking. Using this idea Berera and colleagues built a model involving only four additional fields and no mass corrections. The researchers then compared the model’s observational predictions with constraints on inflation derived from Planck satellite measurements of the cosmic microwave background radiation and found good agreement between the two. The presence of radiation during inflation has been known from very earlier work by Berera and colleagues to lower the tensor-scalar ratio. This model realises this result in a compelling first principles model, thus accommodates the possibility for a tensor mode anywhere from the present upper bound to a value extremely below that.
The latest research is published in Physical Review Letters and was highlighted by the journal as an Editors' Suggestion, which recognises its important contribution to the field.
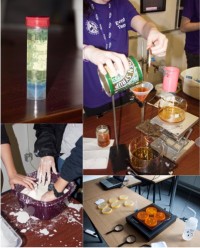
On Saturday 26th September the School took part in Door Open Day with two popular exhibits and a successful recruitment day.
Institute for Condensed Matter and Complex Systems once again threw open the doors to its laboratories for Doors Open Day 2016. Visitors were able to see how complex fluids are studied using rheology, watch food based capsules being made and see the group’s new confocal microscope in action. In the foyer, younger visitors made slime and lava lamps, learning about non newtonian fluids and interfacial phenomena. A popular favourite, a large bucket of corn flour in water dramatically illustrated shear thickening. Well away from the corn starch, highlighted the biophysics research of the group, including an interactive simulation of bacterial population dynamics. A team of 12 PhD students and postdocs from the ICMCS ran the event and talked enthusiastically to visitors about their work.
PP4SS (Particle Physics for Scottish Schools) had successful display in the JCMB cafe with good in visitor numbers.
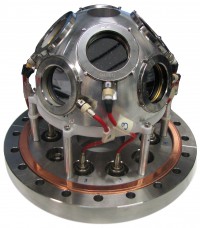
A new experiment carried out in the underground Gran Sasso Laboratory of the Italian National Institute for Nuclear Physics (INFN) could help scientists uncover the origin of some rare elements in the universe.
Nuclear fusion reactions provide the source of energy that makes stars shine. Like gigantic alchemists of the cosmos, they also create new elements from the hydrogen and helium left over by the Big Bang.
Understanding the complex pattern of reactions that leads to the abundances of elements and isotopes that we observe today requires detailed models of stellar evolution and extensive laboratory experiments on individual nuclear reactions. For some time, astronomers have been puzzled by anomalous abundances of rare isotopes of carbon, nitrogen, and oxygen in the atmospheres of some stars and in tiny grains that reach the Earth inside meteorites. In particular, the amount of oxygen-17 – a rare, heavier form of the oxygen we breathe – observed in some red giants and massive stars can provide important clues on the mixing processes that occur inside these stars and bring to their surfaces the debris of nuclear reactions. By measuring the tiny particles released by the fusion of hydrogen and oxygen-17, the study – published in the journal Physical Review Letters – reveals that oxygen-17 is destroyed twice as fast as previously expected: a result that may help reconcile the puzzling abundances observed in stellar atmospheres and stardust grains.
The experiment, which took place at the LUNA (Laboratory for Underground Nuclear Astrophysics) accelerator of the Gran Sasso Laboratory, detected the feeble signals of the fusion reaction with unprecedented precision. Such studies can only be performed in the absence of radiation from space, and the shielding provided by the 1.4km of rock under the Gran Sasso mountain is ideal to suppress the background induced by cosmic rays.
LUNA is an international collaboration of about 40 scientists from the INFN (Italy), the Helmholtz-Zentrum Dresden-Rossendorf (Germany), MTA-ATOMKI (Hungary) and the School of Physics and Astronomy of the University of Edinburgh (UK).
The UK team, led by Professor Marialuisa Aliotta, played a key role in the planning and execution of the experiment and provided the detectors and related instrumentation.
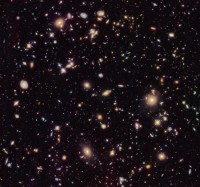
An international team of astronomers, led by Edinburgh, has gained a new view of the evolution of distant galaxies. Their study focuses on a region of the Universe previously studied using the deepest observations of the Hubble Space Telescope.
The team has traced the previously unknown abundance of star-forming gas and dust over cosmic time. This provides new insights into what is known as the golden age of galaxy formation - approximately 10 billion years ago. The new observations of a well-studied area of sky, known as the Hubble Ultra Deep Field, were taken with the ALMA (Atacama Large Millimetre/submillimetre Array) telescope, located high in the Atacama Desert in Chile. The resulting images are significantly deeper and sharper than those from previous surveys undertaken at these long wavelengths. Astronomers have used their findings to show for the first time how the rate of star formation in young galaxies is closely related to their total mass in stars, which is assembled from previous star-formation events.
The findings will be published in in the Monthly Notices of the Royal Astronomical Society.
The first Hubble Ultra Deep Field images, pioneering deep-field observations at optical wavelengths, were captured with the NASA/ESA Hubble Space Telescope and published in 2004. Hubble was refurbished in 2009 and since then, it has captured near-infrared images. The result is the deepest view of the Universe to achieved date, revealing early galaxies that existed less than a billion years after the Big Bang. However, Hubble cannot reveal the emission from young stars that is absorbed and then re-emitted by clouds of interstellar gas and dust, within which the youngest stars form. To do this, astronomers have had to await the advent of ALMA, which for the first time enables the warm glow of dust-enshrouded star-formation activity to be imaged with a depth and clarity comparable to Hubble imaging. The team, led by the University of Edinburgh, used ALMA to obtain the first deep, homogeneous image of the entire Hubble Ultra Deep Field region. This enabled them to clearly match the galaxies detected by ALMA with objects already known from the Hubble imaging. This showed clearly, for the first time, that the mass of stars already existing within a galaxy is the best indicator of how quickly stars are forming in the very distant Universe. The team was able to detect virtually all of the high-mass galaxies and very little else.
According to researchers, the new ALMA results imply a rapidly rising gas content in galaxies as we look back further in time. This is likely the root of a remarkable increase in star formation rates during the peak of galaxy formation some 10 billion years ago. The results are the first in a series of planned observations of the distant Universe using ALMA. Astronomers hope the forthcoming program of surveys will complete their view of the galaxies in the Hubble Ultra Deep Field.