STFC funds for a new Centre for Doctoral Training (CDT) in Data Intensive Science have been awarded to a joint bid from University of Edinburgh, University of Glasgow & University of St Andrews. Fully funded studentships for Home/EU students are available to start in September 2017; applications open now.
We are creating a new Centre for Doctoral Training (CDT) in Data Intensive Science, pushing forward key areas of Astronomy, Particle Physics, Solar Physics and Nuclear Physics, while contributing to the creation of a new generation of data scientists. This is based on our long-standing strengths in the core science areas; our track record of innovation and leadership in applying and advancing data science technologies; and our proven record in running other physics CDTs, as well as an established geographically distributed shared Graduate School.
The key feature of the programme are:
- Four PhD year programme with integrated training.
- 6 month industrial / commercial placement.
- Fully funded 48 months studentships available to suitably qualified candidates to cover stipend and fee at Home/EU rate.
"In Edinburgh we have long been keen on how to extract science from big datasets - especially in cosmology, and in particle physics - using innovative information technology. We are excited to be part of this new initiative", Andy Lawrence, Regius Professor of Astronomy, The University of Edinburgh .
"
Understanding the vast flow of data that characterises our society calls for new methodologies and algorithms. We are excited by the opportunity of applying the knowledge developed in our disciplines to these new challenges.", Luigi Del Debbio, Professor of Particle Physics and Head of Institute for Particle and Nuclear Physics.
For enquiries and how to apply see: Enquiries
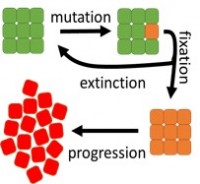
Epidemiologists have long observed that the life-time probability of cancer varies greatly among different organs from which cancers originate. A new study in Science and a Perspective article shed a new light on this problem.
According to the prevalent hypothesis, cancer occurs when alterations of the genetic material cause cells to proliferate in an uncontrolled way. These alterations arise due to “intrinsic” processes such as random errors during DNA replication, and “extrinsic” factors such as chemical mutagens and ionizing radiation. A recent Science paper attempted to estimate contributions from these different processes. Using epidemiological data from 69 countries, researchers Cristian Tomasetti, Lu Li, and Bert Vogelstein (Baltimore) found a strong correlation between the logarithm of the life-time number of cellular divisions in a given tissue and the logarithm of cancer incidence rate in the same tissue. They also combined data from cancer genome sequencing projects and epidemiological studies and showed that mutations due to replication errors account for 2/3 of all mutations found in cancer.
In the Perspective article, Martin Nowak (Harvard) and Bartlomiej Waclaw (Edinburgh) show that although these results are very interesting, there is a huge gap in our understanding what actually contributes to cancer risk.
“Given what we know about cancer biology, the correlation between the risk of getting cancer and the number of stem cell divisions is not that surprising” - says B. Waclaw – “What is more surprising is that cancer risk increases slower than linearly with the number of cell divisions”.
To find out what a theoretical relationship between cancer risk and cellular divisions should be, researchers considered a mathematical model (see the picture). The model shows that cancer risk is the product of the probabilities of cancer initiation and progression. Cells in normal tissues are often divided into “compartments” of size a few to a hundred cells. Initiation requires a single cell to become “neoplastic”: to acquire a mutation that causes the cell to produce more offspring than normal cells do. The population of this neoplastic cell must then take over the entire compartment before it can spread to surrounding tissues. This process, called “fixation”, is stochastic and sometimes neoplastic cells die out. Depending on the number of mutations required to produce the first neoplastic cell, mathematical modelling predicts a linear or faster-than-linear relationship between risk R and the number of cell divisions D, R ~ D^a, where a is larger or equal to 1. However, epidemiological data shows that the risk is a sublinear function of D, and a is approximately 0.5.
The discrepancy between the model and reality means that there must be additional factors that lower cancer initiation rate in tissues with larger number of divisions. For example, additional “checkpoints” may be present in such tissues that need to be deactivated to enable cancer progression. Tissue geometry may also play a role in reducing the initiation rate in tissues with higher proliferative potential. Researchers are now working on mathematical models that would take these factors into account.
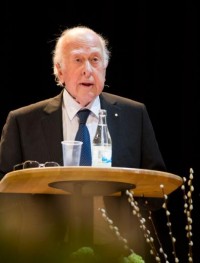
Professor Emeritus Peter Ware Higgs, Nobel Laureate in Physics 2013, has received the Royal Commission for the 1851 Exhibition Medal for his outstanding contribution to physics. The award was made at a Presidential Dinner on Tuesday 28th March 2017 by Princess Anne, the Princess Royal, at the Armourer’s Hall in the City of London.
It is only the third time the Medal has been awarded since its introduction in 2006. The first was awarded to His Royal Highness The Duke of Edinburgh on the fortieth anniversary of his Presidency. The second was presented to Sir Alan Rudge on his retirement as Chairman after eighteen years of service to the Commission. The Commissioners were unanimous in their decision to award the third to Professor Higgs.
Professor Peter Higgs
Peter Higgs held, as a postdoctoral researcher, a Royal Commission for the 1851 Exhibition Senior Studentship from 1953 to 1955. He spent the first year at King’s College London where he worked on what would now be described as theoretical chemistry. He spent his second year of 1954-1955 at the University of Edinburgh at the Department of Natural Philosophy. He remained in Edinburgh for the subsequent year of 1955-1956 as a Senior Research Fellow. He then returned to London, spending time at Imperial College and University College, until his appointment as a Lecturer in the Tait Institute of Mathematical Physics Mathematical Physics in 1960.
Professor Higgs went on to further his studies at Edinburgh and in the 1960s, he developed the theory of the Higgs Field and Higgs Mechanism. He was elected a Fellow of the Royal Society in 1983 and a Fellow of the Institute of Physics in 1991. He retired in 1996, becoming Professor Emeritus at the University of Edinburgh. In 2013, he received the Nobel Prize in Physics, jointly with François Englert, for “the theoretical discovery of a mechanism that contributes to our understanding of the origin of mass of subatomic particles”; a discovery that was confirmed by the ATLAS and CMS experiments at CERNs Large Hadron Collider.
The Royal Commission for the 1851 Exhibition
The Commission was originally founded by Prince Albert to organise the Great Exhibition of 1851 and then use its profits to “increase the means of industrial education and extend the influence of science and art upon productive industry”. With over 6 million visitors to the exhibition there was substantial profits which were used to purchase 87 acres of land in South Kensington stretching from Kensington Gore to Cromwell Road. Here they aided the establishment of the Victoria and Albert Museum, the Science Museum, the Natural History Museum, the Royal Albert Hall and Imperial College as well as the Royal College of Art and the Royal College of Music.
The Commission has a long history of supporting scientists and engineers for research, development and design through its awards programmes, which have been running since the 1890s. It has a total annual disbursement of £2.5m a year and, in addition to Professor Higgs, the Commission has funded twelve other scientists that have gone on to receive Nobel Prizes, including Sir James Chadwick, Ernest Rutherford and Paul Dirac.
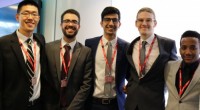
The University of Edinburgh team of physicists went on to win third place in the final gaining a prize of £250 and beating a number of business teams from universities across the UK. A real coup for the School of Physics and Astronomy the University of Edinburgh.
The IBM Universities Business Challenge (UBC) Worldwide is the world’s longest established undergraduate simulation-based competition designed to develop employability and enterprise skills.
Facilitated by Susan Bird (Careers Consultant, School of Physics and Astronomy), the School submitted two teams, one team making the semi-final in Edinburgh. After a keynote introduction from Shelagh Green, (Director, Careers Service), the teams did a series of intensive, timed business simulations culminating in a 60 second innovation pitch.
The Physics team – students Imran Marwat (team leader), Fidel Elie, Ziyi Zhang, Adamos Spanashis and Brandon Christman – not only won 2nd place at the semi-final and went on to win third place in the final - but also won Best Business Idea on the day, beating 19 other teams from other UK universities.
The School funded the team’s travel & accommodation expenses to the London final, the team was mentored by Mike Ross from Standard Life Investments & supported by the Careers Service – a good example of how we work with Schools & industry partners to support the employability and professional development of our science students.
Here’s how team member Ziyi Zhang reflected on the Challenge:
“Thank you for being at the competition and supporting us throughout this experience. I realized studying physics not only made us “book smart” but also taught us critical and logical thinking which can be applied beyond academics”
"Many congratulations to Imran, Fidel, Ziyi, Adamos and Brandon for a truly impressive result in this prestigious competition. It's a great achievement by everyone in the team and is a clear demonstration that the skills they've developed through their time at university have applicability far beyond physics and astronomy." Judy Hardy, Director of Teaching
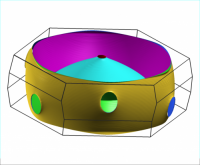
Prof. Graeme Ackland at the School of Physics has received significant EPSRC funding for electronic structure calculations using density functional theory.
The award comes through the United Kingdom Car-Parrinello Consortium (UKCP) collaboration, which has been expanding for 25 years since it was established in 1991 to fund Physics and EPCC to write the first parallel computing density functional theory code. Ackland is the only ever-present co-investigator. UKCP is one of the most prolific collaborations in UK Science, publishing 106 papers in 2015 including Edinburgh-led studies on advanced titanium alloys and the physics of high pressure hydrogen.
Since UKCP began, Density Functional Theory has established itself as the standard method for solving the many-body quantum mechanics of electrons in solids and liquids. It provides a way to calculate a full range of material properties for substances, including properties which are impractical to measure and for materials which have yet to be synthesized.
The 4-year grant is worth over £5M, split 80% for supercomputing at UoE's ARCHER facility and 20% staff costs.
The project will provide resources for School researchers Graeme Ackland, Andreas Hermann, Ingo Loa, Miguel Martinez-Canales to continue their studies on high pressure materials, thermoelectrics, planetary interiors and fundamental understanding and interpretation of Raman, infrared, X-ray and neutron scattering experiments carried out within CSEC.
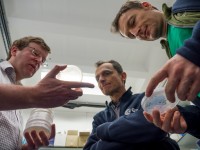
European astronauts have arrived in Edinburgh to have training from scientists at the University. The visitors from the European Space Agency will undergo training in astrobiology to understand how to collect samples during planetary missions to minimise contamination and how to collect appropriate samples that can be used to search for habitable conditions elsewhere and maybe even life.
They will be given lectures in the subject and carry out laboratory work, in addition to using Arthur's Seat in the city centre to learn about geology and how to select samples for further study.
Their training will include how to search for signs of life in rocks, how to take samples with minimal risk of contamination, and how to analyse geological samples in the lab.
Taking part are Mathias Maurer, who joined ESA in 2010 and is scheduled to complete his basic astronaut training this year. His experience includes spending 16 days underwater to test hardware and experiments for the International Space Station, as well as testing exploration strategies and tools for future missions to Mars.
He will be joined by Pedro Duque, who has worked on many space missions with ESA and NASA, and qualified for one of the first European long-term missions to the International Space Station. His spaceflight experience includes a nine-day mission on the Space Shuttle Discovery, and the 10-day Cervantes mission on the International Space Station.
Their visit forms part of the ESA's Pangaea course, which is designed to provide European astronauts with introductory and practical knowledge of Earth and planetary geology.
The course is intended to prepare astronauts to effectively partner planetary scientists and engineers in designing exploration missions.
The course also aims to give astronauts a solid knowledge in the geology of the Solar System by learning from leading European scientists.
Professor Charles Cockell of the School of Physics and Astronomy, and head of the UK Centre for Astrobiology, leads the ESA astronaut training in astrobiology and geomicrobiology.
Professor Cockell said: "We are really excited to be sharing our knowledge and expertise with European astronauts, in a visit which puts us at the forefront of future human exploration missions beyond Earth and introduces Europe's new astronauts to science relevant for their future work."
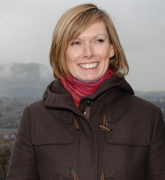
Professor Annette Ferguson has won a Friedrich Wilhelm Bessel Research Award from Alexander von Humboldt Foundation in recognition of her research into the histories of nearby galaxies.
Scientists and scholars, internationally renowned in their field, who in the future are expected to continue producing cutting-edge achievements, which will have a seminal influence on their discipline beyond their immediate field of work, are eligible to be nominated for a Friedrich Wilhelm Bessel Research Award. Award winners are honoured for their outstanding research record.
As part of the award, Annette will spend 6 months at the Leibniz Institute for Astrophysics in Potsdam collaborating with scientists on galactic archaeology projects.
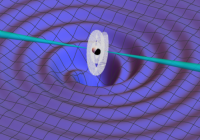
Near the end of the last millennium, our Universe was discovered to be undergoing an accelerated expansion. The physics underlying this acceleration, however, remains one of the Cosmos's darkest secrets. It could be attributed to a new "Dark Energy" force that fills the Cosmos or the presence of the Cosmological Constant predicted by Einstein. But for the two decades since this discovery, there has also been the possibility the acceleration is due to a change in Einstein's Theory of General Relativity. In particular, high hopes were assigned to an extension by a new constituent of the Universe that shares similar properties to the Higgs field.
Researchers at the Royal Observatory in Edinburgh have now discovered that if this field existed it should change the speed of Gravitational Waves from the speed of light, unlike Einstein's theory where these speeds agree. If the speeds did agree, the change in gravity would have messed up the good agreement with the largest structures we observe in our Universe. A difference between the speeds is already at odds with indications from indirect measurements. But with the advent of Gravitational-Wave astronomy marked by the recent detection in the Laser Interferometer Gravitational-Wave Observatory (LIGO), the clear measurement of the speed of Gravitational Waves can now be realised, and so unravel this darkest secret of our Universe.
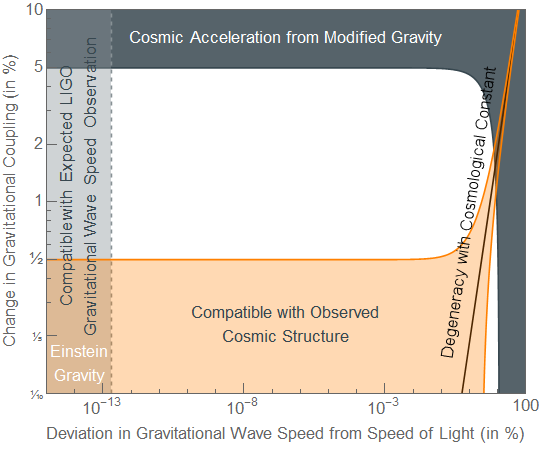
Agreement between the speeds will be strong evidence for the Cosmological Constant that Einstein once considered his biggest blunder. With the new observing runs of the LIGO and VIRGO experiments in 2017, the crucial observation is expected in time for the 100th anniversary of Einstein's Constant.
Lucas Lombriser, one of the authors, says: “Last year’s direct gravitational wave detection has opened up a new observational window to our Universe. It is like being able to hear when one was only seeing. Our results give an impression of how this new sense will guide us in solving one of the most fundamental problems in physics.”
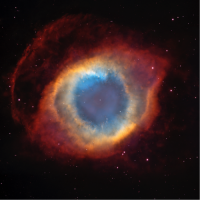
A long-standing puzzle on the origin of stardust recovered from meteorites has finally been solved thanks to the identification of the effect of a nuclear reaction in the composition of stardust grains.
During their lifetime, stars with masses four to eight times the Sun’s blow off their outer layers generating a nebula around them full of stardust and grains. The chemical composition of such dust and grains reveals important clues on the nuclear processes that have contributed to their formation.
The Solar System is believed to have formed from such a nebula. And while most of the original dust was destroyed to make up new rocks and planets, including the Earth, a small fraction survived the destruction process. This special dust, recovered from meteorites, can be used to trace the evolution of the nebula from which the planets were born and to understand the physical processes inside the stars where the grains formed. But tracing the grains to specific types of stars turned out to be surprisingly difficult.
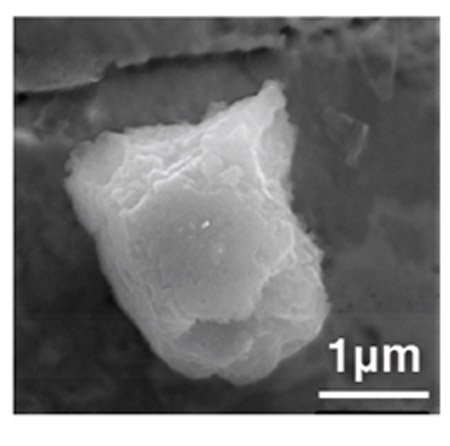
While intermediate mass stars (roughly six times heavier than the Sun) are seen by infrared telescopes to produce huge amounts of dust, however, grains recovered from the Solar System meteoritic record so far did not seem to match the composition expected from these stars.
A new study, led by Maria Lugaro from Konkoly Observatory (MTA-CSFK) and published in Nature Astronomy, solves this problem by identifying, in the make-up of some meteoritic stardust grains, the effect of the nuclear reactions that occur in these particular stars.
The breakthrough was possible thanks to experiments carried out at the Laboratory for Underground Nuclear Astrophysics (LUNA). LUNA observed that the probability for the fusion of protons and 17O (a heavier type of the oxygen we breathe) to occur is twice as large as previously thought (Bruno et al., Physical Review Letters, 117, (2016) 142502). This effect is clearly observed in some stardust grains, resolving the mystery of the missing grains from intermediate-mass stars.
LUNA is currently the only underground accelerator worldwide specifically devoted to the study of nuclear reactions of astrophysical interest. The facility is hosted by the Laboratori Nazionali del Gran Sasso of the Italian Institute for Nuclear Physics (INFN) and is located under more than one kilometer of rocks.
Maria Lugaro says: “The long-standing question of the missing dust was making us very uncomfortable: it undermined what we know about the origin and evolution of dust in the Galaxy. It is a relief to have finally identified this dust thanks to the renewed LUNA investigation of a crucial nuclear reaction.”
“The results of this study prove once again the importance of precise and accurate measurements in the laboratory of the nuclear reactions that take place in stars – says Prof Marialuisa Aliotta, who led the UK team of LUNA for the PRL study – It is a great satisfaction to know that we have contributed to solving a long-standing puzzle on the origin of some stardust grains.”
LUNA is an international collaboration, involving about 40 scientists from 14 institutions in Italy, Germany, Hungary and the UK. The Collaboration Spokesperson is Prof Paolo Prati, from the University of Genova, Italy.
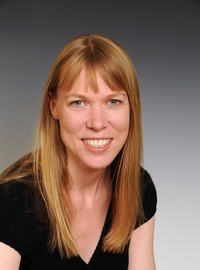
The Institute for Astronomy's Prof. Catherine Heymans has been awarded the 2017 George Darwin Lectureship by the Royal Astronomical Society. The Lecture is given annually, on a topic in astronomy, cosmology or astroparticle physics.
Professor Catherine Heymans is a world-leading observational cosmologist who is well known for her innovative and ground-breaking work in the field of weak gravitational lensing. In recognition, she holds a Personal Chair at the University of Edinburgh. She is the principal investigator for two successive major research grants from the European Research Council that support her team’s research on the Dark Universe and Beyond Einstein Gravity Theories. Since completing her PhD in 2004, Prof. Heymans has co-authored over 100 well-cited articles in peer-reviewed journals. She is a founding member of the Young Academy of Scotland. Professor Heymans is devoted to promoting the public’s understanding of her research. She has appeared in a wide range of events, at numerous Science Festivals, and has written a number of articles for the popular Institute of Physics science magazine 'Physics World'. Her TEDx talk on the Dark Side of the Universe has had over 8000 views on YouTube showing that she is an accomplished public speaker. For these reasons, Professor Heymans is awarded the George Darwin Lecture.