A spacecraft has revealed the first section of what is set to be the largest 3D map of the Universe ever made.
The Euclid space telescope – orbiting one million miles from Earth – has produced a piece of its cosmic map containing some 14 million galaxies and tens of millions of stars in our own Milky Way. Despite its vast scale, the section accounts for just one per cent of the full map that Euclid will produce during its six-year mission.
The mission – launched in July 2023 – is led by the European Space Agency and a consortium of more than 2,000 scientists from 16 countries, including researchers from the University of Edinburgh.
Map section
The newly released map section – 208 gigapixels in size – is composed of 260 observations made by Euclid’s powerful cameras in Spring of this year.
Researchers will use data gathered by the mission to shed light on two of the biggest mysteries in the Universe: dark matter and dark energy. Dark matter – which does not reflect or emit light – is thought to make up around 80 per cent of all the mass in the Universe and binds galaxies together. Dark energy is a mysterious phenomenon that is pushing galaxies away from each other and causing the expansion of the Universe to accelerate. Unlike gravity, which draws objects together, dark energy appears to drive cosmic objects apart at an increasingly rapid rate, experts say.
Edinburgh expertise
Astronomers from the School of Physics and Astronomy are leading on two of the Euclid mission’s key research areas, including analysis of data relating to so-called gravitational lensing. The phenomenon – which occurs when a galaxy causes the path of light to bend around it – produces tiny changes in the images of galaxies, which can be used to map the distribution of dark matter in space and how it has evolved over time. Edinburgh also hosts Euclid’s UK Science Data Centre, which is processing huge amounts of data generated throughout the mission for scientists to analyse worldwide.
Professor Andy Taylor (School of Physics and Astronomy) who leads the UK’s Euclid data analysis team and the Euclid gravitational lensing data analysis, said:
This map of a large chunk of the sky is amazing, and shows Euclid's unique capacity to make high-resolution images of the Universe over such large areas. This is essential for Euclid's mission to understand dark matter and dark energy, but also provides astronomy, and the public, with an unprecedented clear view of the Universe.
Image gallery
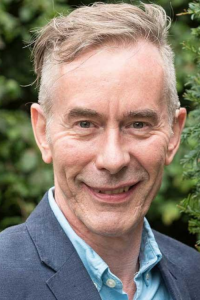
Colleagues are saddened by the loss of physicist Professor Ian Shipsey, who passed away on Monday 7 October.
Members of the School of Physics & Astronomy in Edinburgh are deeply shocked and saddened by the sudden death of our colleague and friend, Professor Ian Shipsey FRS, Head of the Physics department at the University of Oxford.
Professor Shipsey had many connections with the University of Edinburgh.
After studying physics at Queen Mary in his native London, he studied for his PhD at the University of Edinburgh. His thesis title was Measurement of the two gamma decays of neutral K-mesons, using data from the NA31 experiment at CERN.
He then moved to the USA, working at Syracuse and then Purdue before returning to the UK to take up a professorship at Oxford in 2013.
Ian was a valued colleague and collaborator to many of us in Edinburgh. He had remarkable energy and a great love for physics, bringing new ideas, strong leadership and valuable advice to every situation. He understood the importance of collegiality in science, always making time for a friendly and personal conversation when we were attending the same meetings.
Ian was a member of the International Advisory Panel for the Higgs Centre in Theoretical Physics. He was an active collaborator with Edinburgh physicists and astronomers, as was a member of research collaborations including the ATLAS experiment at the LHC at CERN and the Rubin LSST project, working with the particle physics groups and Wide-Field Astronomy Unit.
Ian visited Edinburgh on several occasions over the last decade.
Ian became profoundly deaf in his late-20s as a side effect of medical treatment and later became an early adopter of cochlear ear implants. In 2014, he presented a general interest seminar to the School on the physics and his experience with the implant, a recording of which can be found below.
Our thoughts are especially with his wife, Professor Daniela Bortoletto, also professor in physics at the University of Oxford and a collaborator with Edinburgh physicists, their daughter and family.
Image gallery
PhD students from 31 countries attend training in Peebles, Scotland.
The 2024 European School of High Energy Physics is taking place this autumn at Peebles, Scotland. This event brings together PhD students from across Europe and beyond to study fundamental particle physics through a series of lectures, discussion sessions and group projects.
Participants will have the opportunity to learn from world-leading experts, including Edinburgh physicists Professor Sinead Farrington and Professor Neil Turok. Dr Alan Walker will provide insights into the life and work of Professor Peter Higgs. There are also courses on science communication and outreach, with training designed to support participants in their future studies and research.
The school rotates between different host countries, and is hosted in the United Kingdom this year for the first time since 1998, taking place between 25 September and 8 October.
Organised by the European Organisation for Nuclear Research (CERN), the 2024 school is supported by a local organising committee comprised of representatives from eight UK universities and research institutes, chaired by Dr William Barter from the School of Physics and Astronomy’s Institute of Particle and Nuclear Physics. Sponsorship is provided by the School of Physics and Astronomy at the University of Edinburgh and the Science and Technology Facilities Council (STFC), enabling broader participation from students who might not otherwise be able to attend.
This year the school brings together students from 31 countries, with 47% of attendees women.
Image gallery
Scientists working on the Short-Baseline Near Detector (SBND) at Fermi National Accelerator Laboratory have identified the detector’s first neutrino interactions.
Edinburgh contribution to neutrino detection
Students and post-doctoral staff at the School of Physics and Astronomy are one of the largest UK groups contributing to the experiment.
The Edinburgh team members have made significant contributions to the construction of the SBND cathode with innovative wavelength-shifting foils to enhance light collections, as well as to the understanding of the cosmic-ray tracking, photon detection and trigger systems of the detector before and after its start. Edinburgh scientists were also responsible for key items of the software infrastructure of the experiment getting it ready for the detector running.
Neutrino Detectors
The SBND collaboration has been planning, prototyping and constructing the detector for nearly a decade. The detector was built by an international collaboration of 250 physicists and engineers from Brazil, Spain, Switzerland, the United Kingdom and the United States. SBND will play a critical role in solving a decades old mystery in particle physics.
SBND is the final element that completes Fermilab’s Short-Baseline Neutrino (SBN) Program which includes the ICARUS and MicroBooNE detectors. All of the detectors are types of liquid-argon time projection chambers, and each contributes to the development of this particle detection technology for the long-baseline Deep Underground Neutrino Experiment (DUNE).
Neutrinos and the Standard Model
The Standard Model is the best theory for how the universe works at its most fundamental level. But despite being a well-tested theory, the Standard Model is incomplete. And over the past 30 years, multiple experiments have observed anomalies that may hint at the existence of a new type of neutrino.
Neutrinos are the second most abundant particle in the universe, but are difficult to study because they only interact through gravity and the weak nuclear force, meaning they hardly ever show up in a detector. Neutrinos come in three types, or flavours: muon, electron and tau. Perhaps the strangest thing about these particles is that they change among these flavours, oscillating from muon to electron to tau.
Scientists have a pretty good idea of how many of each type of neutrino should be present at different distances from a neutrino source. Yet observations from a few previous neutrino experiments disagreed with those predictions, which means there could be more than the three known neutrino flavours.
The Short Baseline Neutrino Program at Fermilab will perform searches for neutrino oscillation and look for evidence that could point to this fourth neutrino.
Beyond the hunt for new neutrinos
In addition to searching for a fourth neutrino, SBND has an exciting physics program on its own.
Because it is located so close to the neutrino beam, SBND will see 7,000 interactions per day, more neutrinos than any other detector of its kind. This large data sample will allow researchers to study neutrino interactions with unprecedented precision. The physics of these interactions is an important element of future experiments that will use liquid argon to detect neutrinos.
Whenever a neutrino collides with the nucleus of an atom, the interaction sends a spray of particles careening through the detector. Physicists need to account for all the particles produced during that interaction, both those visible and invisible, to infer the properties of the ghostly neutrinos. With the detector located so close to the particle beam, it’s possible that the collaboration could see other surprises.
One of the biggest questions the Standard Model doesn’t have an answer for is dark matter. Although SBND would only be sensitive to lightweight particles, those theoretical particles could provide a first glimpse at a ‘dark sector’.
Prof Andrzej Szelc, SBND physics co-coordinator based at the School of Physics and Astronomy said:
So far ‘direct’ dark matter searches for massive particles haven’t turned anything up. Theorists have devised a whole plethora of dark sector models of lightweight dark particles that could be produced in a neutrino beam and SBND will be able to test whether these models are true.
These neutrino signatures are only the beginning for SBND. The collaboration will continue operating the detector and analysing the many millions of neutrino interactions collected for the next several years.
Image gallery
The data gathered provides unprecedented insights into the structure and composition of the Milky Way.
The VISTA (Visible and Infrared Survey Telescope for Astronomy), a European Southern Observatory (ESO) facility in Chile, has successfully mapped over 1.5 billion objects in the Milky Way.
Over the course of 13 years, the VISTA Variables in the Vía Láctea (VVV) survey and its extension, the VVV eXtended survey (VVVX), observed the central regions of the Milky Way. Beginning in 2010, these surveys required 420 nights of observation to capture approximately 200,000 images, monitoring over 1.5 billion celestial objects and generating 500 terabytes of scientific data. This represents the largest volume of data ever collected for an ESO observational project.
Notable discoveries
The data gathered provides unprecedented insights into the structure and composition of the Milky Way, the galaxy that contains our own Solar System. Notable discoveries include:
- Globular clusters: the oldest objects in our Galaxy.
- Hypervelocity stars: stars expelled from the Galaxy by the central supermassive black hole.
- Galactic windows: clear views through interstellar dust and gas to the other side of the Galaxy.
- RR Lyrae variable stars: the oldest known population in the centre of the Galaxy.
- Brown dwarf stars and binary floating planets: unique celestial objects that enhance our understanding of stellar and planetary formation.
UK role on VISTA
The University of Edinburgh is one of 18 UK universities which form part of the VISTA Consortium who work closely together with scientists from the Science and Technology Facilities Council through its team at the UK Astronomy Technology Centre (UK ATC) to play a role in the development and delivery of the telescope to the ESO.
Edinburgh involvement
The Wide-Field Astronomy Unit (WFAU) in the University of Edinburgh’s Institute for Astronomy curates and publishes the VVV/VVVX data, along with that from the other public surveys conducted with the VISTA telescope, in its VISTA Science Archive (VSA).
The WFAU Director, Prof Bob Mann said:
Members of the WFAU team – led by Dr Nick Cross – have been working closely with the VVV/VVVX consortium since their survey started. The VSA provides a long-term home for their survey data and many additional data products derived by the consortium, ensuring that their vast, and wonderfully rich, dataset will remain accessible to astronomers. Sky survey data has a scientific longevity, and researchers will be extracting exciting science from the VVV/VVVX dataset for many years to come.
Image gallery
Research could have implications on the emulsification and stability of new materials.
Physicists and mathematicians have turned their attention to a fascinating observation that has intrigued scientists and cocktail enthusiasts alike: the mysterious way ouzo, the anise-flavoured liquor, turns cloudy when water is added.
The research, which involved experts from the University of Edinburgh, Loughborough University and Nottingham Trent University, has resulted in a new mathematical model that offers insights into the spontaneous formation of microscopic droplets and how they can remain suspended in a liquid for a long time.
Revealing the maths taking place in the glass could have far-reaching implications, such as the creation of new materials, especially in fields such as pharmaceuticals, cosmetics, and food products, where the stability and distribution of microscopic particles are critical.
When water is added to ouzo, microscopic droplets form which are a result of the anise oil separating from the alcohol-water mixture. This causes the drink to turn cloudy as the droplets scatter light. The emulsification - the suspension of well-mixed oil droplets in the liquid - is something that requires a lot of energy in other systems and foods, but in the case of ouzo, it happens spontaneously. What’s also surprising is how long these droplets, and the resulting cloudiness, remain stable in the mixture without separating, especially when compared to other food emulsions.
By mixing alcohol, oil, and water in varying proportions, the researchers were able to observe phase separation and measure key properties like surface tension.
They used this data and a statistical mechanical modelling method known as ‘classical density functional theory’ to develop their mathematical model.
This model has been used to calculate a phase diagram that details the stable combinations of the ouzo ingredients.
Image gallery
Detector explores weaker dark matter interactions than ever searched for before.
Exploring weakly interacting massive particles
Figuring out the nature of dark matter, the invisible substance that makes up most of the mass in our universe, is one of the greatest puzzles in physics. New results from the world’s most sensitive dark matter detector, LUX-ZEPLIN (LZ), have narrowed down possibilities for one of the leading dark matter candidates: weakly interacting massive particles, or WIMPs.
LZ hunts for dark matter from a cavern nearly one mile underground at the Sanford Underground Research Facility in South Dakota. The experiment’s new results explore weaker dark matter interactions than ever searched before and further limit what WIMPs could be.
The scientists involved in this latest work have noted that the detector and analysis techniques are performing even better than expected.
LZ uses 10 tonnes of liquid xenon to provide a dense, transparent material for dark matter particles to potentially bump into. The hope is for a WIMP to knock into a xenon nucleus, causing it to move, much like a hit from a cue ball in a game of pool. By collecting the light and electrons emitted during interactions, LZ captures potential WIMP signals alongside other data.
What is dark matter?
Dark matter, so named because it does not emit, reflect, or absorb light, is estimated to make up 85% of the mass in the universe but has never been directly detected, though it has left its fingerprints on multiple astronomical observations. We wouldn’t exist without this mysterious yet fundamental piece of the universe; dark matter’s mass contributes to the gravitational attraction that helps galaxies form and stay together.
International collaboration
LZ is a collaboration of roughly 250 scientists from 38 institutions in the United States, United Kingdom, Portugal, Switzerland, South Korea, and Australia; much of the work building, operating, and analysing the record-setting experiment is done by early career researchers.
At the School of Physics and Astronomy, Professor Alex Murphy and Dr Sally Shaw lead a group of researchers contributing to many aspects of LZ, including neutron backgrounds and searches for other dark matter candidates such as axions. Sally served as LZ’s Physics Coordinator from 2022 to earlier this year, overseeing most of the data-taking and leading a substantial part of the analysis for the new results.
Dr Sally Shaw said:
This result was only possible due to many excellent physicists working very hard together, despite spanning most of the world's time zones! Doing science on this scale is a team game, and the LZ team has done a brilliant job once again delivering world-leading dark matter constraints.
The collaboration is already looking forward to analysing the next data set and using new analysis tricks to look for even lower-mass dark matter.
Image gallery
Congratulations to Dr Cyrielle Opitom who has been appointed a member of the RSE Young Academy of Scotland.
The Royal Society of Edinburgh (RSE) Young Academy of Scotland (YAS) scheme brings together young professionals from all areas of academia, business, third sector organisations and public life, to work together to address the most challenging issues facing society in Scotland and beyond.
Forty eight new members have just been appointed, who will join YAS’s existing members in realising its mission to achieve transformative societal change through citizenship, innovation, collaboration, evidence, and leadership.
Dr Cyrielle Opitom is a Chancellor’s Fellow, based in the School’s Institute for Astronomy. Dr Opitom’s research and expertise focuses on studying small bodies of the solar system and in particular comets. She uses and helps to develop the latest astronomical instrumentation to investigate the composition of comets and to understand what they can teach us about the history of the solar system. Cyrielle is also involved in projects aiming at developing astronomy in Africa.
Festival goers at the World of Music, Arts and Dance (WOMAD) were treated to a feast for the mind last weekend as Edinburgh physicists Professor Victoria Martin and Dr Alex Hall gave talks at the World of Physics pavilion.
WOMAD festival started in 1980 as the brainchild of musician Peter Gabriel, and takes place near Malmesbury, Wiltshire. The annual festival showcases musicians and performers from around the globe, and since 2016 has also hosted a range of physics events including talks, workshops, demonstrations, and panel discussions.
Professor Martin from the Institute for Particle and Nuclear Physics gave a talk celebrating the life and work of the late Professor Peter Higgs. She commented:
It was a great honour to be at WOMAD to talk about Peter Higgs and his legacy to physics and to our world. I'm sure Peter would have very much appreciated the spirit of the festival of bringing people together to celebrate the arts, science and culture from all over the globe.
Dr Alex Hall from the Institute for Astronomy gave a talk on the Euclid space telescope, showcasing its incredible imaging power and explaining the fundamental physics of dark matter and dark energy that the mission aims to shed light upon.
Dr Hall said:
It was a privilege to be invited to speak at WOMAD. Having science events alongside literature, art, and music exposes people to different ideas and perspectives that they may not usually encounter and is one of the reasons this festival is so special.
Both talks were given to a capacity crowd of several hundred and were very well received by the engaged and captivated audience.
The talks were sponsored by CERN & Society Foundation.
Image gallery
Students on the MSc degree in Astrobiology and Planetary Sciences have been sharing their knowledge and understanding about life on other planets with a video games company.
Auroch Digital, an independent game development company based in Bristol, is creating the video game ‘Mars Horizon 2: The Search for Life’, whose players will run a space agency, investigate the solar system and collect evidence of life.
The collaboration, which also involved astrobiology staff, included a number of online meetings and a visit from game developers to the University. Students carried out research and made scientific suggestions to inform in-game scenarios.
A tour of lab facilities conducted during the visit involved a demonstration of chemical reactions, the viewing of samples including rocks, minerals, organisms and fossils, and a guide to the ‘Mars chamber’, which reproduces the pressure, temperature and atmospheric composition of Mars to see how various materials and processes behave under those conditions.
Students on the interdisciplinary MSc degree in Astrobiology and Planetary Sciences work to understand the nature of life and whether we might find it elsewhere in the universe. They build on their knowledge of physics, chemistry, biology and geosciences to answer fundamental questions about living matter, how it forms, varies and evolves in concert with planets and stars, and how it is distributed across time and space.
An estimated 3.2 billion people worldwide play video games, making this one of the most popular forms of entertainment. With the steady rise in popularity of gaming over the past few years, there may be further opportunities for collaboration with scientists.
Madeleine Landell, a student on the MSc Astrobiology and Planetary Sciences degree commented:
Collaborating with Auroch on Mars Horizon 2 has been an incredible opportunity. It has both given us astrobiology students a glimpse into the creative and meticulously researched process that goes into videogame development, as well as allowing us to think differently about advances in space science. The Auroch team's dedication to preserving as much real science as possible in the video game was really impressive. They weren't afraid to get into the semantics of molecular biology and geochemistry in their pursuit of a scientifically accurate yet exciting game. We've left this collaboration with renewed excitement about how our current astrobiological research could contribute to the future of space exploration. We really look forward to the release of Mars Horizon 2.
John O’Donnell, Lead Game Designer on Mars Horizon 2 said:
We had so much fun collaborating with the students of the MSc programme. It was a privilege to see and hear about their work and have their influence on the game’s vision and authenticity. The students helped us research many what-if scenarios of life in our solar system and because of their diverse backgrounds this took the game in interesting new directions.