Congratulations to Dr Ross Galloway on who has been awarded the 2022 Institute of Physics Marie Curie-Sklodowska Medal and Prize in recognition of work in developing, using and communicating research-based approaches to active student learning in physics and other disciplines.
The Institute of Physics Marie Curie-Sklodowska Medal and Prize is awarded to those who have made distinguished contributions to physics education.
Dr Ross Galloway’s achievements cover two areas of higher education: firstly, the use, promotion and evaluation of the flipped classroom approach, in which the transfer of knowledge occurs remotely, usually online, and contact with the teacher involves student-centred activity; secondly the use of PeerWise, a revolutionary approach to teaching in which students generate content themselves, including questions and problems to be solved by other students.
Both these areas of work are pioneering in the UK and have been influential in encouraging take up in many other universities. These approaches have also been expanded to developmental sessions in disciplines beyond physics.
Flipped classroom teaching was introduced in 2011 by Dr Galloway as part of the Edinburgh team. He has carried out many evaluation studies, initially focusing on outcome metrics (e.g. using the Force Concept Inventory to evaluate conceptual understanding). More recent studies have included qualitative aspects of student/instructor behaviour to determine what really works and to disperse the false dichotomy between traditional and active learning, instead focusing on which aspects of student activity result in the most valuable learning.
Dr Galloway was part of the first wave of UK engagement with PeerWise from 2010. The distinctive Edinburgh approach was their extensive use of a research-informed scaffold, to allow students to understand the unfamiliar task of writing problems rather than solving them, to build their confidence and to highlight what is effective assessment. He led the deployment of those scaffolding activities and made many improvements over several years. He also carried out research on how students used PeerWise and determined that, when properly supported, students can generate high-quality questions, indicating deep learning and sophisticated understanding of the physics beyond that typically obtained in more passive approaches.
In addition to physics education research, Dr Galloway provides input to the Institute of Physics Degree Accreditation Committee, and provided a major contribution to the revised accreditation approach which is less content-focused and places more emphasis on degree outcomes relating to students' conceptual understanding of physics, problem solving and broader skills.
Machine learning provides a new way of revealing the physical quantities behind the images observed by telescopes.
Machine learning (ML) is a novel method which uses artificial intelligence (AI) to make predictions with data. Artificial intelligence means using computers to do complex tasks, such as recognizing objects from pictures and playing chess. A lot of applications of ML appeared in many different fields of industry and research in recent years. It not only speeds up the process by efficiently dealing with a great amount of data, but also leads to new methods and new findings.
International collaboration
An international group including experts in astronomy research from the University of Edinburgh, Universidad Autonoma de Madrid (Spain), Sapienza University (Italy) and experts in machine learning models from the EURA NOVA company (Belgium), have started a collaboration to provide new and simple ways to infer important physical quantities of clusters of galaxies from multiwavelength images. Recently, they focused on accurate estimates of the mass of galaxy clusters from the Planck satellite microwave images. This study is published in the latest issue of Nature Astronomy.
Measuring galaxy mass
Galaxy clusters are the most massive object that ever formed in our universe, so precisely measuring their masses has very important meanings for many different astronomy studies. In order to obtain its mass from observed images, astronomers have to first process the image by excluding fore/background objects and removing the noises, then different assumptions have to be made to derive the mass from binned image quantities, such as profiles. These assumptions normally oversimplify the state of the real cluster, therefore the mass derived with such methods doesn’t agree with the true mass. This difference is referred to as bias. Furthermore, the whole process is very time-consuming and the clusters have been handled one by one.
Machine learning
The machine learning method proposed by this group overcomes all these problems and can directly get the cluster mass from observed images. And it is very fast - in just a few seconds, over 1000 observed cluster masses are provided. The machine learning model is based on a type of deep learning algorithm known as the Convolutional Neural Networks (CNN), which can get the most important features of an image to connect with a defined quantity. A perfect tool for this task. However, this involves training the model with over 10 thousand images from numerical simulations of galaxy clusters, of which the group used the results from The THREEHUNDRED project, hosted in Universidad Autónoma de Madrid (UAM). They verified the cluster mass from the machine learning model has no bias and has a very small scatter around the true cluster mass. Applying this model to the images observed by the Planck satellite, they provided bias-free cluster masses of over 1000 galaxy clusters.
PhD student Daniel de Andres from UAM has completed most of the work on this project. The paper’s co-leading author, Dr Weiguang Cui, from the School’s Institute of Astronomy commented:
These results as very exciting, and machine learning is proving to be a useful tool which will help us understand our Universe.
Art work produced following a summer collaboration will be exhibited in an Edinburgh gallery this October.
A collaboration involving art students and physics researchers has led to the creation of a series of art work, sculptures and digital graphics, showcasing a range of physics and astronomy concepts.
The exhibition will take visitors on a visual journey across a range of physics research which takes place within the School of Physics and Astronomy. It includes art work depicting the formation and structure of the universe; the application of physics in the study of microorganism growth; and in the use of supercomputers for computationally intensive tasks such as quantum mechanics. It also features portraits of students and researchers.
Three Edinburgh College of Art students collaborated with 20 researchers during the 10 week summer project, brought together by communications and outreach colleagues.
School of Physics and Astronomy organisers commented:
This is a great opportunity to help convey the work which takes place behind lab doors, to communicate complex ideas, and to share some fascinating concepts in a visual format.
The exhibition, called ‘Fusion: Physics X Art’ takes place at Whitespace Gallery in Newington, Edinburgh from Friday 14 to Tuesday 18 October 2022.
The DART - OPTiK team will observe NASA’s asteroid deflection mission from their base in Kenya.
The DART - OPTiK team, which includes a collaboration of researchers from the University of Edinburgh, Science and Technology Facilities Council (STFC), Technical University of Kenya and the Turkana Basin Institute (TBI), set up a portable telescope at the TBI base in Ileret, Kenya and have been taking observations over the past month.
Tonight, they will observe NASA’s Double Asteroid Redirection Test (DART) as the spacecraft launched in November 2021 deliberately collides with its target asteroid. The moment of impact will only be visible from countries around the Indian Ocean, with the dark skies of Ileret making it an excellent vantage point from which to observe this unique event.
DART Mission
The purpose of NASA’s first ever planetary defence test is to demonstrate that the path of an asteroid through space can be changed using a ‘kinetic impactor’: a spacecraft that is deliberately crashed into the asteroid at high speed.
Although the target asteroid is not a danger to Earth, it is expected that the technology being tested can be used, should an asteroid on an Earth-impacting trajectory ever be discovered.
The test will be carried out on a binary asteroid system, which includes a large asteroid (Didymos, 780m diameter) and a smaller moon (Dimorphos, 163m) orbiting it. The DART spacecraft will hit Dimorphos at 14,000 mph, slightly changing its orbit around Didymos. The impact time will be 00:14 BST on Tuesday 27 September, with live images from the spacecraft broadcast online by NASA.
Next steps
The DART- OPTiK team, as well as astronomer teams across the globe, will monitor the orbit of the moon to measure how effective the 'kinetic impactor' experiment was. The team will observe the aftermath of the collision over the coming weeks by monitoring the asteroid’s moon using its lightcurve, and obtain more detailed follow up data using larger telescopes at established observatories in Chile, as part of an international effort.
A related European Space Agency (ESA) mission, Hera, will launch in the coming years to the same asteroid to study the effects of the collision in detail.
Kenyan collaboration
The DART - OPTiK telescope will also be used to do astronomical tests to discern whether the site at Ileret is suitable for a more permanent observatory in the future. Working with the Technical University of Kenya, the Kenyan Space Agency, and the Kenya Optical Telescope Initiative, the team hope to strengthen capacity for local astronomy and facilitate new research in the region.
The UK’s first Hyperloop test track has been built by students from the University’s HYPED team, at the King’s Buildings.
Hyperloop technology
Touted as a future fifth mode of transport, Hyperloop technology would see pods carry people and cargo at high speeds through steel tubes between terminals. Hyperloop is designed to use less energy: the pods are magnetically levitated just above the track, thereby reducing the slowing effects of drag. It is also envisaged that pods would travel through near-vacuum tubes where most of the air has been removed to reduce aerodynamic resistance and energy use.
Popularised by entrepreneur Elon Musk, Hyperloop’s advocates believe it could be a more sustainable alternative to short and medium haul air travel – potentially achieving a London to Edinburgh commute in 50 minutes.
Edinburgh’s HYPED team, which includes students from the School of Physics and Astronomy, is dedicated to advancing Hyperloop, and is the leading university team developing the technology in the UK. It provides a testing ground for engineering students to tackle the technical challenges of building a functioning Hyperloop pod from scratch.
Test track
The new test track is a key missing piece of the jigsaw for HYPED. Each year the team competes in international competitions alongside other top university teams from around the world – which often requires them to test their pod on the track on competition day itself, without having the chance to properly trial it beforehand.
The track is a 100-metre-long aluminium rail, travelling in a straight and smooth line over a concrete foundation, designed by HYPED’s student engineers and located on Max Born Crescent, between Murchison House and FloWave.
The rail was manufactured with support from the University of Edinburgh’s Estates Department, the College of Science and Engineering, and the schools of Engineering, Physics and Astronomy and Informatics.
Prototype pods, which range from three to five metres in length, will potentially reach up to 90 mph over the track, once testing begins in November. Installed just a short walk from the HYPED lab in Hudson Beare building, the track will provide the team with an accessible way to test and validate their design ideas and prototypes for the first time.
Activities and outreach
After constructing their first pod prototype in 2017, HYPED has grown into a thriving and diverse society of around 130 students from across the University. Before the pandemic, they were the only UK-based team to qualify for the annual SpaceX Hyperloop Pod Competition in California for several years running until the competition ceased in 2019.
In more recent years, they co-founded European Hyperloop Week alongside other top European university teams Delft Hyperloop, Swissloop and Hyperloop UPV – as an annual gathering to bring those working on the technology together, to compete, network, and share ideas.
The team also runs outreach events to inspire school children and the members of the public about Hyperloop technology, and STEM subjects more widely. This includes an upcoming ‘Gender Minorities in STEM Workshop’ for students in Scottish secondary schools in November, where they hope to showcase the track.
HYPED President, School of Engineering student Gregory Dayao explained:
We will use the track as a space to validate our technical designs and demonstrate our vision of Hyperloop to the Edinburgh community. By engaging with the local community, we want to increase awareness of Hyperloop technology and inspire young students to pursue early careers in STEM. The test track is a leap forward for the society to ensure HYPED provides an innovation-centred ecosystem for University of Edinburgh students to develop and generate value towards Hyperloop development.
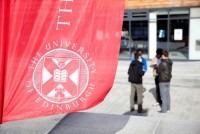
Come along to our Open Days to find out more about studying at the University of Edinburgh and the School of Physics & Astronomy
Our Open Days will take place on Saturday 8th October and 5th November 2022. The School of Physics & Astronomy is located in the James Clerk Maxwell Building, in the King’s Building’s Campus. At the School of Physics & Astronomy you will get the chance to find out more about our Undergraduate degree programmes, meet academics and students, and tour our physics labs.
Please check the University of Edinburgh Open Day pages to find out more and book your place.
Astronomers have taken the first image of an exoplanet – a planet beyond our solar system – with the James Webb Space Telescope.
This is the first image of an exoplanet with the James Webb Space Telescope (JWST), as well as the first image of an exoplanet at wavelengths longer than 5 microns.
An exoplanet is a planet beyond our solar system. Learning about exoplanets teaches us new information about how the universe works.
This image is of exoplanet HIP 65426b. It is a young, giant planet. As a young planet, it is still very hot, and hence can be detected by its glow in the infrared.
The image captured shows the exoplanet as seen in different bands of infrared light: purple shows the NIRCam (near infrared camera) instrument’s view at 3 micrometers, blue shows the NIRCam instrument’s view at 4.44 micrometers, yellow shows the MIRI (mid infrared instrument) view at 11.40 micrometers, and red shows the MIRI instrument’s view at 15.50 micrometers. These images look different because of the ways that the different filters capture light. The small white star in each image marks the location of the host star, HIP 65426. The JWST team used instruments known as coronagraphs to block out the light of the host star to reveal the planet.
Prof Beth Biller, based at the School’s Institute for Astronomy, is the co-principal investigator of a James Webb Space Telescope Early Release Science Programme:
This is the first of hopefully many exoplanet images from the JWST. With JWST's unprecedented sensitivity, we can detect and characterise both young giant planets like HIP 65426b, but also potentially young analogues to Saturn and Neptune as well!
Dr Trent Dupuy and PhD student Pengyu Liu from the School were also involved in the JWST Early Release Science Programme as part of a large international collaboration involving 100+ scientists.
Image gallery
Polymer physics modelling predicts interactions in cancer genomes.
An interdisciplinary team of physicists and biologists, including Dr Chris Brackley from the Institute for Condensed Matter and Complex Systems at the University of Edinburgh, and Dr Lisa Russel and Dr Daniel Rico from Newcastle University, used polymer physics based simulations to make predictions of the three-dimensional organisation of chromosomes near to sites of incorrect repair.
Their model predicts which enhancer sites will contact the genes in different cancer cells. The lab-based team can then perform experiments where these sites are targeted, with the aim of reversing the disease state. Not only are the simulations a useful tool for helping direct the experiments, but they also give important insight into the biological and physical processes which lead to the loops forming.
Gene regulation
The chromosomes in our cell nuclei are long fibres made up from DNA and proteins. One mechanism through which genes are regulated is via changes in the three-dimensional organisation of the chromosomes. For example, often, to activate a gene, sites on the chromosome called ‘enhancers’ have to be brought into physical contact with the gene, folding the chromosome into a loop.
Many cancers arise when the chromosomes are broken, and then incorrectly repaired. For example, the broken ends of two different chromosomes can become joined together; genes near to the join can find themselves in contact with enhancers they would not normally encounter. This leads to chemical modification of the chromosome near these genes (chemical ‘marks’ known as H3K4 tri-methylation, or H3K4me3 are deposited). These ‘oncogenes’ then become constantly active, promoting continuous cell division and cancer.
Dr Lisa Russel, Cancer Biologist at Newcastle University said:
Not only do the simulations allow us to understand how the H3K4me3 domains translocate, but they suggest sites on the DNA which can then betargeted in experiments that aim to reverse the disease.
Published work
The work is part of a set of three papers from the same groups of authors which feature of the cover of the July 2022 edition of Genome Research. Together, these works show that H3K4me3 broad domains are associated with regulatory elements of cell identity genes, called super-enhancers, that are ‘hijacked’ to interact with other genes in cancer cells; this is associated with the disappearance of the H3K4me3 domains from cell identity genes, and their appearance over oncogenes. The work showed that the regulation of H3K4me3 broad domains is associated with leukemogenesis and suggests that the presence of these structures might be used for epigenetic prioritisation of cancer-relevant genes.
Science–art collaboration
The teams also worked together with multimedia science artist Dr Dimple Devadas, who produced a series of artworks inspired by the projects. One of the artworks was used on the journal cover, and the others appear in an online exhibition Science as Genome art, showcasing this coming together of cancer biology, polymer physics, and art.
Observations from the James Webb Space Telescope have revealed the most distant galaxy so far.
A team of astronomers led by the University of Edinburgh have discovered what they believe is the most distant galaxy ever observed.
The galaxy has a redshift of 16.7. Redshift is used to measure distances in the cosmos, and describes the way light coming from an object has been stretched by the expansion of the universe to redder wavelengths. The higher the redshift number, the more distant it is and the earlier it is being viewed in cosmic history.
The galaxy discovered is called CEERS-93316, and is 35 billion light-years away. It is taken from a project called the Cosmic Evolution Early Release Science (CEERS) Survey which is taking some of the first observations with the James Webb Space Telescope (JWST).
The colour image of CEERS-93316, recently featured by BBC News, was made by Edinburgh undergraduate physics students Sophie Jewell and Clara Pollock, who are undertaking summer projects in the Institute for Astronomy, funded by summer studentship bursaries from the School of Physics and Astronomy.
The same team have also published an analysis of the first spectroscopic data from the JWST, led by Leverhulme Fellow Adam Carnall. This work confirms the vast potential of JWST spectroscopy for performing detailed studies of the earliest galaxies, such as CEERS-93316. This work was recently reported on by Science News.
James Webb Space Telescope (JWST)
The JWST is the successor to the Hubble Space Telescope, was launched in December 2021 and first collected images from its home at the second Sun-Earth Lagrange point in June 2022. JWST observations will include the first stars, formation of the first galaxies, our own solar system, and will tell us more about the atmospheres of potentially habitable exoplanets.
PhD student Callum Donnan who is based in the Institute for Astronomy and involved in the project commented: “We’re using a telescope that was designed to do precisely this kind of thing, and it’s amazing.”
Next steps
Observations from the JWST have yet to undergo full spectroscopic examination. This process will reveal the spectra of galaxies and will explain how the light (originally visible wavelengths) has been stretched into the infrared. The spectroscopy will also reveal the chemical composition of objects.
Callum Donnan explains: “We can look at the colours in our galaxy in a broad sense, and it’s quite blue, which suggests a younger stellar population. But it’s not blue enough that this galaxy is made up of metal-free stars”.
The discovery by the Edinburgh team will be short lived however – astronomers expect to find ever more distant objects via the images from the JWST over the coming weeks, months and years.
Image gallery
The world’s largest and most sensitive dark matter experiment has come to life and is delivering results, moving a step closer to offering clues about one of the biggest mysteries of the Universe.
The LUX-ZEPLIN Dark Matter Experiment (LZ), based at the Sanford Underground Research Facility in South Dakota, US, has gathered its first result – showing the experiment is successfully operating as designed.
What is LUX-ZEPLIN?
LZ is intricately and innovatively designed to find direct evidence of dark matter – a mysterious invisible substance thought to make up most of the mass of the Universe. Dark matter is particularly challenging to detect, as it does not emit or absorb light or any other form of radiation. The LZ detector will try to capture the very rare and very faint interactions between dark matter and its 7-tonne liquid xenon target.
The international project led by the Department of Energy’s (DOE) Lawrence Berkeley National Laboratory (Berkeley Lab) has now announced the detector is running as hoped after years of careful set-up. The tests show that LZ already is the world’s most sensitive dark matter detector, with plans to collect about 20 times more data in the coming years. After the successful start, full-scale observations can begin with the hope of finding the first direct evidence of dark matter.
The legacy of ZEPLIN
LZ involves an international team of 250 scientists and engineers from 35 institutions from the US, UK, Portugal, and South Korea. The UK team, funded by the Science and Technology Facilities Council (STFC), consists of more than 50 people from the universities of Bristol, Edinburgh, Imperial, Liverpool, Oxford, Royal Holloway, Sheffield and UCL and STFC’s Rutherford Appleton Laboratory.
Many of these UK groups came from the ZEPLIN programme, which developed the liquid xenon technology for dark matter searches at STFC’s Boulby Underground Laboratory. The UK ZEPLIN groups then joined the LUX experiment in the USA in 2012 and started designing LZ around that time.
Professor Alex Murphy who leads Edinburgh’s group on the project said:
“It’s great to see all these years of development, from ZEPLIN through LUX and now LZ come together. It’s been a huge team effort”
Hunting the dark matter
LZ is the largest and most sensitive experiment searching for dark matter particles. These theorised elementary particles interact with gravity – which is how we know about the existence of dark matter in the first place – and possibly through a new weak interaction too. These first results place strong constraints on one possibility for what dark matter might be, Weakly Interacting Massive Particles (WIMPs). WIMPs would be expected to collide with ordinary matter – albeit very rarely and very faintly. This is why very quiet and very sensitive particle detectors are needed for WIMP detection.
How does it work?
At the centre of the experiment is a large liquid xenon particle detector maintained at -100oC, surrounded by photo-sensors. If a dark matter particle interacts with a xenon atom, and produces even a tiny amount of light, the sensors will capture it. In order to see these rare interactions, the team have had to carefully remove all natural background radiation from the detector materials first.
By operating around a mile underground, LZ is shielded from the cosmic rays that bombard experiments located at the surface of the earth. The detector and its cryostat sit inside a huge water tank to protect the experiment from particles and radiation coming from the laboratory walls.
Finally, the team made sure that the liquid xenon itself is as pure as possible by carefully removing a key contaminant through a complex years-long process.
Prof Murphy commented: “With LZ running so well, we should be able to really test the WIMP model for dark matter. There are many other possibilities for what dark matter might be too, several of which are a real focus for the Edinburgh team. It’s a really exciting time for the field.”
IMAGE INFORMATION
Title: LZ Surface Lab
Credit: Matthew Kapust, Sanford Underground Research Facility