The UK Centre for Astrobiology (UKCA) at the University of Edinburgh has officially launched the UKCA Harding Essay Competition 2025, inviting undergraduate and postgraduate students across the UK to take part.
This annual competition offers a unique platform for students to explore topics that connect science, culture, and philosophy. Participants are encouraged to share their insights and original ideas, with the opportunity to have their work published on the UKCA website and win exciting prizes.
The competition is open to students from all disciplines — whether aspiring journalists, scientists, or creative writers. "This is a chance for students to make their voices heard and engage with some of the biggest questions about life, the universe, and our place within it," the organisers said.
Entries must be submitted by 31 July 2025 via email to UKCA-info [at] ed.ac.uk. Full details, including competition guidelines are available on the UKCA webpage.
Extreme conditions create stable compounds, offering planetary insights.
Carbon, nitrogen, and hydrogen are crucial elements in the solar system. They are essential for the origins of life. The simplest molecules from these elements are methane (CH₄) and nitrogen (N₂). These typically do not react under normal conditions, but on Saturn’s moon Titan, interactions suggest otherwise. To understand how these reactions might happen deep within planets, researchers examined these molecules under extreme pressures and temperatures.
During the research, mixtures of methane and nitrogen were squeezed using a tool called a diamond anvil cell, to simulate high pressure environments, with fascinating results.
At pressures above 7 GPa (~70,000 atm) two new compounds (CH₄)₇(N₂)₈ and (CH₄)₅N2 formed. Remarkably, when pressure exceeded 160 GPa, the methane and nitrogen molecules broke apart, forming a new material with carbon, hydrogen, and nitrogen atoms bonded tightly together. Surprisingly, this new material remained stable even after the pressure was removed.
The research team also discovered that heating methane-nitrogen mixtures to over 1400°C at 40 GPa caused it to break down into powdered diamond and ammonia.
The findings can be found in a paper published in Angewandte Chemie by PhD student Hannah Shuttleworth. The project was led by colleagues from the School’s Institute for Condensed Matter and Complex Systems (ICMCS): Dr Ross Howie, Dr Miriam Peña-Alvarez and Professor Andreas Hermann.
These discoveries provide new insights into chemical processes potentially occurring in planetary interiors, contributing significantly to our understanding of planetary chemistry.
Dr Ross Howie, ERC Research Fellow said:
This research highlights how pressure alone can be used to induce reactions in seemingly stable systems, which is crucial in mimicking the conditions found within the interiors of planets.
Image gallery
The European Space Agency’s Gaia space telescope will be turned off after eleven years of observing from deep space. A team at the Institute for Astronomy, who have worked with the spacecraft during the entire operational phase, reflect on this milestone, the successes of the mission so far and the work still to be done.
Eleven years in deep space
The European Space Agency’s Gaia spacecraft was launched on 19 December 2013 and has been operating almost continuously for the last eleven years, scanning the sky with its twin telescopes as part of its mission to build a detailed map of the Milky Way. It resides in deep space at the second Lagrange point in the Sun-Earth system (‘L2’), a gravitationally neutral point that is a favourable location for making observations, and which hosts numerous space telescopes. The space environment is harsh, and Gaia has been subject to continuous radiation exposure, solar flares, and micro-meteorite impacts during its lifetime. However, it was built to be resilient, and it has remained operating almost without fault for the entire eleven years – more than twice as long as originally planned. Continual near-realtime monitoring of the health of the onboard instruments has been carried out by a team at the Institute for Astronomy as part of their contributions to the mission data processing.
‘Passivation’
Gaia has now run out of the cold gas propellant that is used to maintain its orientation, and the final science observations were collected in mid January. Since then the spacecraft has been undergoing a series of end-of-life tests to better understand the behaviour of its instruments and the status of numerous components after more then a decade in space. In late March it will be finally turned off, a process referred to as ‘passivation’. This involves first moving it away from L2 and putting it into a heliocentric orbit that keeps it safely away from operational satellites. On the 27 of March the final passivation steps will be carried out – the remaining propellant will be dumped, all electronic components turned off and the onboard software that controls the spacecraft will be deleted.
Work still to do
Although the spacecraft itself will be retired, the on-ground work of processing the raw data, production of the star catalogues and preparation for their release to the scientific community will continue for several years to come. Gaia observations are used to measure the distances to stars by triangulation, and although interim star catalogues have been published over the last few years based on partial datasets, the nature of the calculation means that the final, best accuracy cannot be reached until all the data is available. At the Wide Field Astronomy Unit, part of the Institute for Astronomy, a team works on the detailed characterisation of the scientific instruments, development of the calibration models and the creation of the data processing software. These are crucial inputs for the conversion of the raw data to science-ready catalogues of stars. With each successive publication of data from the mission the entire Milky Way map is redrawn, benefiting from improvements to the instrument models, data processing systems and increased number of data points. Now that the raw science dataset is complete, the production of the final star catalogue can begin. This will be released by the end of 2030.
Dr Nick Rowell, Senior Sky Survey Researcher at the School's Institute for Astronomy said:
The Gaia mission continues to deliver unprecedented insights into the history of our galaxy the Milky Way, as well as many other branches of astronomy. The Gaia space telescope has survived more than eleven years in the harsh environment of space where it has been battered by solar flares, cosmic radiation and regular meteor strikes. It has now run out of propellant and reached the end of its lifetime, so must be put into a disposal orbit where it won't pose a risk to operational satellites. The telescope has delivered huge volumes of data that we will be working hard to prepare and release over the next few years. As yet, only around a quarter of the observations have been included in the data released to the scientific community, and there is a lot of work still to do.
Dr Nigel Hambly Senior Researcher at the School's Institute for Astronomy said:
Gaia has proved to be remarkably resilient - a testament to all the engineers and scientists involved. It is a privilege for our team at the Institute for Astronomy to continue to play a significant role in the mission as we move into the post-operational phase. Preparation of the final data release products for the full 10 year mission will keep us occupied for several years to come and we look forward to further exciting discoveries as the world astronomical community continues to exploit the Gaia data releases.
Image gallery
Congratulations to Professor Richard Ball who has been elected as RSE Fellow.
Professor Richard Ball
Congratulations to Professor Richard Ball who is among the distinguished individuals elected to become Fellows of the Royal Society of Edinburgh (RSE).
Professor Ball works in the area of particle physics theory, the study of elementary particles and their interactions as revealed by experiments at particle colliders such as the Large Hadron Collider at CERN. He has a broad range of interests, from chirality and the Higgs boson, to high energy quantum chromodynamics, and the extraction of precision physics from hadronic collisions using neural networks and machine learning.
Royal Society of Edinburgh
The Royal Society of Edinburgh, Scotland’s National Academy, is an educational charity established in 1783.
New Fellows are elected to the RSE each year through a nomination process and the Fellowship comprises around 1,800 leading experts. The RSE uses its combined knowledge of Fellows to tackle the most pressing issues facing society, provide independent expert advice to policymakers and inspire the next generation of innovative thinkers.
Data sheds light on how mysterious forces shaped the evolution of the Universe.
Q1 Release
The “quick look” (Q1) release of the first survey data from the European Space Agency’s pioneering Euclid satellite – made public today – has led to a flurry of scientific advances that further our understanding of the cosmos.
Launched in July 2023, Euclid is mapping the Universe with unprecedented precision and accuracy. By examining more than one billion galaxies over six years, this groundbreaking space telescope aims to explore two of astronomy’s biggest mysteries: dark matter and dark energy.
Despite covering less than 0.5 per cent of the complete study area, the data is already proving to be a treasure trove for UK scientists.
Researchers from the University of Edinburgh lead the UK’s involvement in the data analysis and host the UK’s Science Data Centre for Euclid.
As a key part of the Euclid Consortium, Edinburgh processes the huge amounts of data from the satellite before it is studied by teams in the UK and across the wider community. This process is already having exciting ramifications for astronomy.
Spectacular discoveries
Euclid has scouted out the three patches of the sky that it intends to, eventually, make the deepest observations of its mission: Deep Field North, Deep Field Fornax and Deep Field South. Put together, these previews of the Euclid deep fields make up the equivalent area of sky more than 300 times the full Moon.
Many of the results using this data have been led by UK based scientists, including discoveries of strong gravitational lensing systems, the characterization of active galactic nuclei (AGN) and the identification of supernovae.
Those results are described in a set of 27 scientific publications, as well as 7 technical papers that describe how this data has been processed by the Science Ground Segment, which receives the initial data from the Euclid satellite and transforms it into data that scientists can work with instantly.
Science Ground Segment
Edinburgh’s leading role in the partnership of seven UK universities which contribute strongly to the Science Ground Segment is instrumental not only to today’s data release, but also more extensive releases in the coming years.
Professor Andy Taylor of the University of Edinburgh, who leads the UK Science Ground Segment and the gravitational lensing data analysis for the mission, said:
These latest results show just how powerful Euclid is for astronomy and how fantastically its data analysis pipeline is working, with such high-quality data over huge areas of the sky. The strong sensing results in particular, led by UK teams, are a huge advance of previous analysis. It has been a lot of hard work over many years to develop, but the results are amazing.
Dr Gordon Gibb, University of Edinburgh and Technical Lead of the UK Science Data Centre, said:
The vast quantity of data collected by Euclid requires considerable infrastructure on the ground to process it. Edinburgh proudly hosts the UK's data centre for Euclid processing, comprising state of the art hardware and an excellent team of software developers and scientists to ensure timely delivery of high-quality data ready for scientific exploitation.
A much larger data release, DR1, is scheduled for October 2026 which will include results about the nature of dark energy.
Image gallery
Breakthrough demonstrates a link between these topological structures to unique hydrogel material properties.
Using a combination of experiments and simulations, researchers from the School’s Institute for Condensed Matter and Complex Systems investigated the material properties of hydrogels made of DNA building blocks, called ‘nanostars’.
The team used experimentally-validated simulations to accurately quantify the internal structure of the gels, and then employed experiments to test simulation-based predictions on how the gels’ elasticity depended on varying DNA concentration.
They discovered that DNA nanostars formed long-lived, interpenetrating networks that had never been seen nor conjectured before. The DNA nanostars created a ‘network-within-a-network’ with loops that interlinked each other and thus presented non-trivial ‘topology’.
The crux of the findings was the demonstration of a link between these complex topological structures and the material properties of the gels. The team also observed similar structures in other network-forming materials, including ice.
The group hopes that their research will pave the way for a new concept in the science of complex fluids, such as gels, pastes, soaps, and other liquids, which fall into the category of ‘topological viscoelasticity’, where the topology of the microscopic network determines its material properties.
Dr Yair Augusto Gutierrez Fosado from the School's Computational Materials Physics research group said:
This significant result enhances our understanding of the connection between the macroscopic mechanical properties of a network, with mathematically rigorous quantities, such as topological invariants. We believe that 'topological viscoelasticity' will be a central theme in rheology of complex fluids in the future.
Image gallery
MSc Astrobiology and Planetary Sciences students visit Boulby Mine, the world's first subsurface astrobiology lab.
Students enrolled on the MSc degree in Astrobiology and Planetary Sciences have literally deepened their knowledge by descending a kilometre underground to explore life in extreme environments.
In December 2024, the students travelled to Boulby Mine, the location of the world's first subsurface astrobiology lab, established by the University of Edinburgh’s UK Centre for Astrobiology (UKCA) in 2013.
The mission to the subsurface began with lectures on using mine facilities as analogues for extraterrestrial environments. The students then completed health and safety training and donned protective gear for the expedition underground.
Inside the mine, students practised sterile sampling methods and investigated salt mineral deposits similar to those found on Mars. They learned about geothermal heat, ionizing radiation, geology, and subsurface microbiology. A highlight was touring a Science and Technology Facilities Council (STFC) laboratory including dark matter and neutrino detectors.
Salma Malaika, MSc Astrobiology and Planetary Sciences student said:
From just a few hours underground, I was able to gain an intuitive feel for what it means to be a researcher in an isolated world, and to truly see the beauty and intricacy behind conducting research in a challenging environment. My passion for the field—and admiration for researchers and staff—has only grown.
Andrew Wright, MSc Astrobiology and Planetary Sciences student commented:
Surrounded by halite crystals, 1000 meters below the surface, few things can compare. Being able to witness the frontier of dark matter and astrobiology research in person was astounding! It was like experiencing another world on Earth and was an experience I’ll never forget.
The immersive activity was part of MINAR (Mine Analog Research) which brings scientists and technologists together to use the Boulby underground laboratory to conduct science and test equipment in support of space exploration. This visit was made possible by the kind support of the STFC and ICL Boulby.
Image gallery
New research reveals that bacteria have evolved to help neighbouring cells after death.
A research team has made the surprising discovery that a type of gut bacteria has evolved to use one of their enzymes to perform an important function after death.
Darwin’s theory of natural selection provides an explanation for why organisms develop traits that help them survive and reproduce.
Because of this, death is often seen as a failure rather than a process shaped by evolution.
When organisms die, their molecules need to be broken down for reuse by other living things. Such recycling of nutrients is necessary for new life to grow.
Now a study led by Durham University and involving researchers from the Universities of Edinburgh and Oxford have shown that a type of E-coli bacteria produces an enzyme that breaks the contents of their cells down into nutrients after death.
The dead bacteria thus offers a banquet of nutrients to the cells that were their neighbours when they were living.
Lead author, Professor Cann from Durham University said:
We typically think of death being the end, that after something dies it just falls apart, rots and becomes a passive target as it is scavenged for nutrients. But what this paper has demonstrated is that death is not the end of the programmed biological processes that occur in an organism. Those processes continue after death, and they have evolved to do so. That is a fundamental rethink about how we view the death of an organism.
Co-author Professor Wilson Poon, from the School of Physics and Astronomy of the University of Edinburgh, inspired the research after posing what he believed were some unanswered questions about why organisms die the way they do.
The researchers assembled and realised they had stumbled across a potentially new area of biology: processes that have evolved to function after death.
Professor Cann said:
One problem remained; we couldn’t work out how an enzyme that functions after death could have evolved. Typically, we think of evolution acting on living organisms not dead ones. The solution is that neighbouring cells which gain nutrients from the dead cells are likely to be clonally related to the dead cell. Consequently, the dead cell is giving nutrients to its relatives, analogous to how animals will often help feed younger members of their family group.”
Co-author Professor Stuart West of the University of Oxford added:
This is like nothing we have observed before – it is equivalent to a dead meerkat suddenly turning into a pile of boiled eggs that the other members of its group could eat.
The finding demonstrates that processes after death, like processes during life, can be biologically programmed and subject to evolution.
Biomolecules that regulate processes after death might be exploited in the future as novel targets to bacterial disease or as candidates to enhance bacterial growth in biotechnology.
Professor Poon suggests that modelling such processes using the tools of statistical physics may also provide design principles for humans as we move towards a more circular economy in which recycling needs to be built in from the beginning.
The study has been published in the journal Nature Communications.
Training events hosted by physicists and astronomers empower Girl Guide leaders to inspire young girls in STEM through hands-on challenges and activities.
In an inspiring collaboration, Girl Guiding leaders are being equipped to bring the wonders of physics to young girls. Through two interactive training events, local Girl Guide Leaders learned how to deliver the exciting challenges and activities that form the ‘I am a Physicist’ badge.
‘I am a physicist’ Girlguiding badge
The ‘I am a physicist’ Girlguiding badge introduces girls to the world of physics in a fun and educational way. The badge is open to all Girlguiding sections and includes activities for the youngest 5 year old Rainbow to the oldest Ranger at age 18.
The challenges
In order to complete the ‘I am a physicist’ Girlguiding badge, girls have to undertake four challenges:
- Experience: engage in simple and fun activities that introduce basic ideas and build confidence in conducting experiments.
- Create: apply these ideas by making or building something practical and imaginative.
- Investigate: undertake experiments to explore the question ‘What happens if…?’
- Meet, visit, community: meet a physicist or visit a museum or science centre where they can see physics in action.
Training events for Girl Guide Leaders
In November 2024 and February 2025, 30 Girl Guide leaders attended workshops hosted by the School of Physics and Astronomy. Led by Dr David Fairhurst, with support from PhD students and staff from the Royal Observatory Edinburgh, these sessions provided leaders with hands-on experience of the badge activities, as well as resources and guidance on delivering them to their local groups.
Each leader left the workshop with a specially curated ‘goodie bag’ from the Royal Observatory Edinburgh, containing fun tools to inspire their girls. The training not only boosted the leaders’ confidence but also equipped them with practical resources to make physics engaging and accessible.
The training sessions received glowing reviews from attendees. One leader shared her enthusiasm:
That was such a fab afternoon, loads of fun and learning. Great pizza and I can't believe the goodie bag. Would recommend to anyone if they do another. Thank you to all who organised.
Broader Impact
The ‘I am a Physicist’ badge was created by the East Midlands branch of the Institute of Physics (IOP) and has already reached over 50,000 participants across the UK and internationally. By fostering curiosity and confidence in physics, the initiative encourages young girls to envision themselves as scientists, engineers, and innovators of the future.
Thanks to the following who made the sessions possible:
- Sue Midgely, Girl Guiding Edinburgh, who provided some funding
- Deborah Phelps, Rolls Royce, Derby, Girl Guiding Nottinghamshire, and former Institute of Physics East Midlands
- Fiona Travers and Abi Ashton, Royal Observatory Edinburgh who provided goodie bag material
- Sammi Bowers, Institute of Physics Scotland, who provided some funding
- Eugénia Delacou, Larissa Palethorpe, Mariam Arif, PhDs student at the School of Physics and Astronomy.
Image gallery
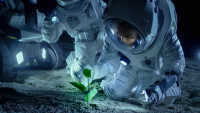
Find out about the School of Physics and Astronomy MSc programmes.
MSc Astrobiology and Planetary Sciences
In this online session, Prof. Charles Cockell will talk about the MSc in Astrobiology and Planetary Sciences. Come along to find out more about programme structure, course options, projects, student experience and career opportunities.
The event will take place on Blackboard Collaborate and a link to join will be sent to those who sign up to attend.
Session details
Date: 19th February
Time: 3-4pm (UK time)
MSc Particle and Nuclear Physics
In this online session, Prof. Christos Leonidopoulos will talk about the MSc in Particle and Nuclear Physics. Come along to find out more about programme structure, course options, projects, student experience and career opportunities.
The event will take place on Blackboard Collaborate and a link to join will be sent to those who sign up to attend.
Session details
Date: 23rd April
Time: 3-4pm (UK time)