European grants success for School researchers
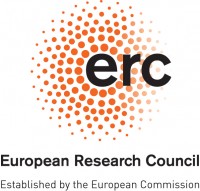
Two School researchers have been awarded European funding to enable them to consolidate their research teams and develop their most innovative ideas.
European Research Council (ERC) Consolidator Grants are awarded to excellent mid-career scientists. This year's recipients include the School's Catherine Heymans and Davide Marenduzzo. You can read about their work below.
Dr Catherine Heymans: Global Lensing Observations To Go Beyond Einstein (GLOBE)
What is the exact nature of our dark universe? It is generally thought that today's universe is filled with a dark energy that is fuelling its expansion. But this conclusion could be a simple misunderstanding of how gravity works on cosmological scales; the universe might not be expanding as we think it is. Dr Catherine Heymans has been awarded an ERC Consolidator Grant to undertake a ground-breaking gravity experiment on some of the largest scales observed in the universe. The project will challenge the most advanced theoretical frameworks, going beyond the theory of Einstein to bring about a revolution in our understanding of gravity and the dark universe. Dr Heymans will call fundamental physics into question by moving gravity experiments out to the largest scales and confronting widely accepted theories with completely new ideas about gravity based on the best available data. The research team will examine the effect gravity has on both the motion of galaxies and light in the same region of space. The evidence collected will be particularly compelling owing to the high quality of the data from the European Southern Observatory. The ultimate goal of the project is to perform a high precision experiment to inspire and confront new theories about how gravity works and thus better understand the dark universe.
Dr Davide Marenduzzo: 3D Cell Physics
The physics of 3-dimensional chromosome and protein organisation within cell DNA and chromosomes are very important biopolymers, as they encode the genetic information which is the unique blueprint of any living organism, be it a bacterium or a human being.
Although we now know in detail the 1-dimensional base pair sequences of many genomes of different organisms, we currently still know very little about how DNA and chromosomes are organised in 3 dimensions inside the nucleus of a living cell. This is of importance for many different reasons. Think for instance of one of your brain cells, and a liver cell in your body. These have exactly the same genetic information in terms of base pair sequence, yet the pattern of genes which are active and inactive is completely different, and this is largely correlated to the 3D structure which the chromosomes adopt. Similarly, during ageing and in some diseases both the global chromosome arrangement and the local structure of chromatin (the DNA-protein complex which is the building block of chromosomes) change, often dramatically. Understanding DNA organisation in vivo is an important task; it is also a very challenging one. To see why, think of the following analogy: if we were to blow up dimensions so that a human nucleus becomes 10m in size, the total DNA length which needs to fit within it would equal 1000 km, yet the region a protein (such as a transcription factor) has to locate accurately, in order to activate the correct gene, would be only about 1mm long.
During this ERC project we will study the biophysics of DNA and chromosome organisation, by means of multiscale computer simulations based on stochastic models developed in our groups and elsewhere. The size of mammalian genomes and complexity of the problem requires very large-scale simulations which exploit the supercomputers at our disposal in Edinburgh and in the UK. Importantly, our simulations are informed by large-scale bioinformatic datasets available publicly and through a network of biological collaborators at Edinburgh and within Europe who will be an important part of the project. At the end of the project we hope to have developed a computer simulation model which can reproduce the organisation of DNA in 3D within bacterial and eukaryotic cells, as well as potentially predict how it changes during development, ageing or disease.