Fresh theoretical understanding of the behaviour of turbulent plasmas could inform potential applications, from tokamak fusion reactors to new understanding of magnetic fields in cosmology. Researchers at the School of Physics & Astronomy have developed a new mathematical description of the energy flow of a turbulent plasma, and how the loss of energy from a plasma can be controlled.
The study, led by Prof. Arjun Berera and PhD student Moritz Linkmann using the ARCHER supercomputer, has led to the first simplified formula to quantify these effects in plasmas affected by magnetic fields. The work also offers new insights into energy flows between fluids and magnetic systems, aiding understanding of how magnetic energy can grow at large scales in a plasma.
Novel insights into turbulent plasma
Recent work at the School of Physics and Astronomy has added novel insights into how the growth, flow and decay of energy in a turbulent plasma can be controlled by the plasma viscosity, the state of magnetic helicity (internal angular momentum and degree of tangledness of the magnetic field) and the state of cross helicity (correlation between the magnetic field fluctuations and the fluctuations of kinetic energy inherent in a turbulent plasma).
A new formula is obtained for understanding the flow of energy out of, and therefore the energy maintained in, a turbulent plasma, which depends on the state of magnetic and cross helicities contained in the magnetic field-fluid system. These results, obtained by a combination of theoretical work and numerical simulations using the ARCHER supercomputer, show how this energy flow can be controlled, leading to the first simplified formula to quantify these effects in magnetofluids. Understanding has also been obtained in how energy flows between the fluid and the magnetic field, adding new insights on how magnetic energy can grow at large length scales in a turbulent magnetofluid. These theoretical results are fundamental steps towards potential practical applications in areas as varied as controlling the plasma in a tokamak fusion reactor and understanding the presence and growth of magnetic fields in galaxies, galaxy clusters and even at the scale of the entire Universe.
This work has come out in a Physical Review Letter and an earlier Physical Review E Rapid Communication. Both figures below contain results from the two publications, all obtained from medium to high resolution simulations carried out on ARCHER.
Results shown in Fig.1 extend the accuracy and extent of detail from previous results in the literature, while results shown in Fig. 2 had been anticipated in terms of qualitative expectations but were never studied systematically before. Their papers have in turn proposed a new way of looking at the problem and by doing so obtained a simple expression derived from the underlying equations that can predict and explain the behaviour seen in these figures, which is the main significant new advance from this work. Their systematic studies have been made possible to a large extent through access to ARCHER, which enabled them to probe a significant section of parameter space.
It has been known for some time that certain correlations between the velocity and magnetic vector fields alter the dynamics of turbulent magnetofluids. What is new from our work is it predicts with a simple expression how the flow of energy out of a turbulent plasma can be controlled based on the viscosity and state of angular momemtum in the magnetic-fluid system. This is a fundamental step toward potential practical applications in areas as varied as controlling the plasma in a tokamak fusion reactor, understanding the presence and growth of magnetic fields in galaxies, galaxy clusters and even at the scale of the entire Universe.'' Arjun Berera
"Much work still needs to be done before quantitative theoretical predictions can be made. Our results are fundamental in the sense that they apply to turbulent magnetofluids far from the boundaries of a containing vessel. Therefore this does not give the full details for specific geometries, such as of a fusion reactor, but our results describe the general behaviour of evolution of the plasma far away from any boundaries, thus are of general applicability to a range of plasmas systems.'' Moritz Linkmann
This work has been published in a Physical Review Letter and an earlier Physical Review E Rapid Communication:
"Magnetic helicity and the evolution of decaying magnetohydrodynamic turbulence"
Arjun Berera and Moritz Linkmann
Phys. Rev. E 90, Rapid Communication, 041003(R) – Published 22 October 2014
https://journals.aps.org/pre/abstract/10.1103/PhysRevE.90.041003
"Nonuniversality and Finite Dissipation in Decaying Magnetohydrodynamic Turbulence"
M. F. Linkmann, A. Berera, W. D. McComb, and M. E. McKay
Physical Review Letters 114, 235001 (2015) – Published 11 June 2015
https://journals.aps.org/prl/abstract/10.1103/PhysRevLett.114.235001
Image gallery
Tributes have been paid to a Nobel Prize-winning scientist and former Principal of the University of Edinburgh, to mark the 50th anniversary of his death.
A wreath has been laid at the grave of Professor Sir Edward Appleton, who is best known for his pioneering work in radio waves, and on the development of radar. Professor Appleton, born in 1892, was Principal of the University of Edinburgh from 1949 until his death in 1965.
Before taking up his post at Edinburgh, he enjoyed a successful career at the University of Cambridge and King’s College London. He is best known for demonstrating the existence of the upper atmosphere – the ionosphere – and researching its effects on radio waves.
During the First World War Professor Appleton served in the army and saw active service. In the Second World War, he was appointed chief civilian scientist, as Secretary of the Department of Scientific and Industrial Research. His work on the ionosphere contributed directly to the war effort in the fields of communications, intelligence and radar development.
Professor Appleton is recognised as playing a pivotal role in establishing the UK as a leader in ionosphere research, in so aiding the development of a radar early-warning system. He was knighted in 1941 and won many awards, including the 1947 Nobel Prize in Physics.
He was drawn to Edinburgh by the University’s status and the appeal of the city. As Principal, he was very involved in administration, including the development of George Square. He founded the Ionospheric Research Group in what was then the Department of Natural Philosophy, and worked enthusiastically until his death aged 72.
The University’s main building in George Square is named the Appleton Tower in his honour, as is the Rutherford Appleton Laboratory research centre in Oxfordshire. He is buried in Morningside Cemetery, Edinburgh.
“Sir Edward Appleton was a pioneering scientist who left an enduring legacy for Edinburgh and beyond. He was a committed, long-serving Principal at the University and it is fitting that we remember his anniversary and the value of his work.” Professor Arthur Trew, Head of the School of Physics & Astronomy, who laid the memorial wreath.
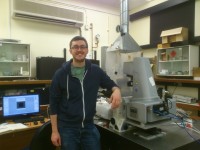
Ben Guy gave an ‘excellent talk, very well judged for the audience and exceptionally well delivered’.
Doing high-quality basic science that also has industrial impact is a tall order for even the most experienced academics. School postgraduate student Ben Guy has not only managed this, but he's also persuaded senior academics and industrial scientists that he is a superb communicator.
Ben works to understand the flow of concentrated suspensions of particles in liquids. Such ‘pastes’ are relevant for ceramics manufacturers because every ceramic product starts life as a paste of refractory particles. The paste needs to flow and deform to order. In traditional pottery, it is moulded by hand. In industry, the paste can be pumped into a mould with complex shape, or extruded (eg into a honeycomb catalyst support), or, in the latest development, 3D-printed into various micro-components. It turns out that the flow of concentrated suspensions with particle sizes in the range of most interest to many industrial applications, 1-10 μm, is not at all well understood.
Ben has discovered that the deformation and flow (‘rheology’) of pastes with particles in the 1-10 μm range is dominated by the ubiquitous phenomenon of shear thickening – an increase in suspension viscosity with applied shear stress when the latter increases beyond a critical value. On the fundamental level, Ben’s data provide evidence for the correctness of a theory of shear thickening recently proposed by Mike Cates and Mathieu Wyart (his collaborator at New York University), which suggests that shear thickening is due to the formation of frictional contacts between particles at high applied stress. Practically, Ben has established a framework for industrialists to ‘navigate’ the complex phenomenology they observe in their real-life materials.
For Ben’s work to make a real impact, he has not only to publish it in scholarly journals, but also communicate it to industrialists directly, translating academic jargon into industrial language and embedding the results in relevant practical contexts.
Every year, Ben's industrial sponsor Johnson Matthey holds a conference where 200 industrial and academic scientists come together to hear their final-year sponsored PhD students present their work, and to look at posters from their earlier-year colleagues.
Ben has already presented two posters previously. This year, he gave a talk on his rheology work to an audience of which some 70% were catalyst chemists. The communication challenge he faced was therefore rather more severe than most of the other speakers, having to bridge between academia and industry, but also between disciplines (physics and chemistry). He succeeded magnificently, winning the prize for the best talk amongst 30 of his peers. Prof. Hugh Stitt, F.R.Eng, one of JM’s scientific consultants, told Ben that he gave an ‘excellent talk, very well judged for the audience and exceptionally well delivered’.
“I am both pleased and surprised to receive this award – industrial scientists and academics really do think differently! The industrial experience has been a challenging but rewarding one and should add an extra dimension to my thesis.” Ben Guy
Ben is sponsored by UK-based international chemicals company and ceramics manufacturer Johnson Matthey (JM).
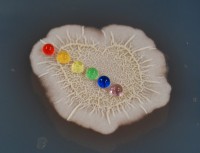
Fresh insights into how bacteria protect themselves – by forming a waterproof raincoat – could help develop improved products to protect plants from disease.
Researchers have discovered how communities of beneficial bacteria form a waterproof coating on the roots of plants, to protect them from microbes that could potentially cause plant disease. Their insights could lead to ways to control this shield and improve its efficiency, which could help curb the risk of unwanted infections in agricultural or garden plants, the team says.
Scientists at the Universities of Edinburgh and Dundee studied the protective film formed by the common soil bacterium Bacillus subtilis. They found it incorporates proteins that change shape as they reach the film surface. This exposes an impervious surface on the protein molecules, enabling them to slot together like a jigsaw puzzle, to protect bacteria underneath. The film is able to repel water – which means other potentially harmful molecules also bounce off. Researchers say that being able to control the production of the biofilm in agricultural products could enable improved protection for plants.
The study, funded by the Engineering and Physical Sciences Research Council and the Biotechnology and Biological Sciences Research Council, is published in Proceedings of the National Academy of Sciences. The team behind the finding plans to research further applications for their discovery.
“Such a controlled shape change in a protein is unusual. This protein only responds in exactly the right way and in the right place. It protects microbes from the outside world, but the ability to control the creation of a water-repellent film has many possible applications.” Prof. Cait MacPhee, School of Physics and Astronomy
Our findings highlight one of the amazing mechanisms that bacteria have evolved to provide protection from changes in their environment. It also demonstrates the advances that can be made when biologists and physicists work together on a problem of mutual interest.” Dr Nicola Stanley-Wall, Division of Molecular Microbiology, University of Dundee
Watch an animation showing how bacteria protect themselves by forming a water-repellent raincoat: https://www.youtube.com/watch?v=hO3HjtJ1TsE
IoP meeting on Nuclear physics and r-process nucleosynthesis
We are pleased to announce an IoP-sponsored half-day meeting on “Nuclear Physics and R-process Nucleosynthesis”, which will take place on Friday May 15th at the University of Edinburgh.
This half-day meeting will bring together nuclear physicists, astronomers, and astrophysicists studying nucleosynthesis during rapid neutron-capture process. It will provide a venue to learn about exciting developments in the field, such as the observation of new elemental abundances in metal-poor stars, r-process models that include increasingly sophisticated treatment of the dynamical evolution of the astrophysical environment, and the opportunities for experiments with r-process isotopes with a new generation of radioactive ion beam laboratories and new equipment (eg the AIDA detector for beta-decays developed in the UK). The event will also be an opportunity to discuss future lines of research and ways to coordinate the efforts of our communities across disciplinary lines.
Invited speakers include: Norbert Christlieb (University of Heidelberg), Giuseppe Lorusso (National Physical Laboratory), Tomislav Marketin (University of Zagreb), and Nobuya Nishimura (Keele University).
Get involved
All members of the UK and international community are welcome to participate, and invited to register before May 5th.
Please register through the meeting website: https://indico.ph.ed.ac.uk/indico/event/iop2015
Those interested in giving a presentation are encouraged to submit a brief abstract by April 26th.
For further information, contact Alfredo Estrade (aestrade [at] ph.ed.ac.uk).
Image gallery
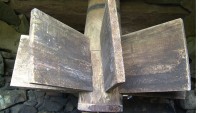
The School is currently showing an exhibition on hydro power in Scotland
The School is currently showing an exhibition in Shetland on hydro power in Scotland together with artist Marianne Greated from Glasgow School of Art. It includes a short film which traces the heritage of small-scale hydro power in Scotland, starting with the horizontal water wheels used for powering small click mills, through to modern micro-hydro plants.
There is also an interactive model water-wheel. Shetland was noted for its horizontal wheels which powered small click mills used by farmers for grinding corn. It is estimated that in the early 19th century there were about 500 such water-powered click mills in Shetland alone and water power was the driving force behind the early Industrial Revolution. Today numerous micro-hydro plants are making a significant power input to the grid and there are still substantial untapped hydro resources in Scotland.
In collaboration with colleagues in Engineering, the School has a research programme that is developing new techniques for mapping untapped hydro resources.
The exhibition, called Revolutions, is sponsored by EPSRC and Historic Scotland.
Image gallery
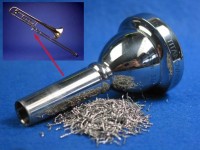
An Edinburgh Team led by Prof Graeme Ackland has discovered that at extreme shear rates, metals can melt: this "shear melting" is well known in colloids and soft solids, but almost unachievable in alloys. For pure titanium shear rates would be well beyond modern machining capability.
Titanium is hard to break. For applications, this is its big advantage, but for manufacturing it is a problem. In a lathe the waste material forms a long unbroken spiral chip which clogs the machine. The goal is to create an alloy that breaks easily in machining, but not in use.
An Edinburgh team led by Prof Graeme Ackland has discovered that at extreme shear rates, metals can melt: this "shear melting" is well known in colloids and soft solids, but almost unachievable in alloys. For pure titanium, shear rates would be well beyond modern machining capability.
To facilitate "shear melting", titanium could be mixed with an element which lowers the melting point. Typically, this would weaken the alloy, so the team chose something that does not dissolve: a rare earth metal (REM). REMs form small inclusions in the alloy under normal conditions, leaving its properties intact, but cutting breaks these inclusions releasing the REM into the alloy. In a feedback effect, cutting releases the rare REM, allowing shear melting which enables further fracture until the chip breaks.
The group of Carsten Siemers in Braunschweig has created the alloy, and in machining tests show that it does indeed break off in small pieces. The company GfE is now looking to develop it commercially.
The most likely applications for the alloy are where we can replace steels (which are heavy) or aluminium (which is soft). One company has already made a batch for use in trial applications in dental tool couplings and mouthpieces for brass instruments. In fact my collaborator in Braunschweig is a trombonist and has tested one of the mouthpieces.
Physical Review Letters paper
The work was supported by the EU "MAMINA" training network, and is reported this month in Physical Review Letters:
"Shear melting and high temperature embrittlement: theory and application to machining titanium". Con Healy, Sascha Koch, Carsten Siemers, Debashis Mukherji and Graeme J Ackland, Physical Review Letters, April 2015
Image gallery
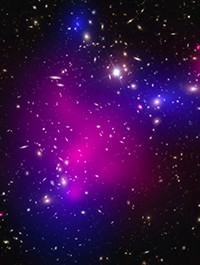
Scientists have furthered their understanding of dark matter, the elusive material that accounts for much of the mass of the Universe. They used NASA telescopes to study how dark matter behaved during cosmic crashes between galaxies in deep space. Each collision took hundreds of millions of years, and is captured as a freeze-frame from a single camera angle.
Their findings show that dark matter interacts with itself even less than was previously thought. It improves scientists’ understanding of the mysterious substance, and helps pinpoint what it might be made of.
“We expected to find that dark matter had minimal interaction with other objects, but we were surprised at how dark and elusive it seems to be." Andy Taylor, Professor of Astrophysics at the Institute for Astronomy, University of Edinburgh, who took part in the study.
Astronomers used observations from the NASA/ESA Hubble Space Telescope and NASA’s Chandra X-ray Observatory to examine 72 large cluster collisions. They studied what happened to their constituent stars, clouds of gas, and dark matter.
They saw that dark matter passed straight through the violent collisions, without slowing down, showing that it does not interact with visible particles, or with itself.
Narrowing down the properties of dark matter will help improve scientists’ models of the Universe.
Further research will examine whether dark matter particles bounce off each other, and will look at collisions between individual galaxies, which are much more common.
Download the Paper
The non-gravitational interactions of dark matter in colliding galaxy clusters
The study, published in the journal Science, was carried out by a collaboration co-led by
the University of Edinburgh and the École Polytechnique Fédérale de Lausanne.
Image gallery
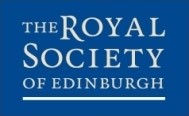
Congratulations to Charles Cockell and Andrew Liddle who have been appointed as Fellows of the Royal Society of Edinburgh, Scotland’s national academy.
"I'm very happy to be recognised by the Society and look forward to helping it in its many roles." Andrew Liddle, Professor of Theoretical Astrophysics at the Institute for Astronomy.
“It's exciting to be elected to the Society as it provides an opportunity to further contribute to both the work of the Society and science in general, particularly the interface between biology and space sciences.”
Prof. Charles Cockell, who leads the Astrobiology group within the Institute for Condensed Matter and Complex Systems
The Royal Society of Edinburgh (RSE)
Established in 1783, the RSE is an educational charity. Unlike similar organisations in the rest of the UK, the RSE’s 1500 Fellows includes people from a wide range of disciplines - science & technology, arts, humanities, social science, business and public service. This breadth of expertise makes the RSE unique in the UK.
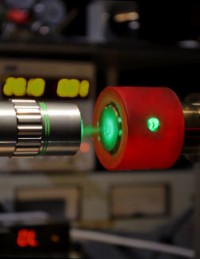
Experiments by researchers in the School's Centre for Science at Extreme Conditions (CSEC) have revealed anomalous behaviour in the melting temperatures of high-pressure dense hydrogen.
As reported in the prestigious "Nature Materials”, the study provides evidence that at above 200 GPa (2,000,000 atm) surprisingly low temperatures (480 K) are required to reach a liquid phase.
As the simplest, lightest and most abundant element of the Universe, hydrogen is of fundamental interest in many fields of physics. At high pressure and low temperatures, hydrogen is predicted to transform to a new type of ordered quantum fluid possessing both superconducting and superfluid properties. The high temperature behaviour is crucial to planetary science as hydrogen is believed to be found in the centre of Jovian planets and to be the source of their exceptionally high magnetic fields. Reaching such conditions in the experimental laboratory, long enough to comprehensively study the material, has been a great challenge in the field of high pressure research. Even high compression at room temperature was thought to be beyond experimental capabilities until recently.
Through new technological breakthroughs in the containment of hot hydrogen in diamond anvil cell experiments, Ross Howie, Philip Dalladay-Simpson and Eugene Gregoryanz at the Centre for Science at Extreme Conditions, provide evidence that after a maximum in the melting curve, the melting temperatures of hydrogen rapidly decrease with pressure, dropping to 480 K at 255 GPa. This consequently results in the lowest melting temperature for any material at such pressures.
Tracking this transformation over a large pressure regime through Raman spectroscopy, the team also discovered a triple point between hydrogen’s solid molecular Phase I, the recently discovered solid mixed molecular-atomic phase IV and the liquid phase.
Remarkably, at pressures above 260 GPa the melting line flattens out, raising many questions about the high pressure, high temperature behaviour of hydrogen at even higher densities. If the melting curve were to increase at higher densities then the phase diagram would markedly resemble what is observed in the rest of the group I elements in the periodic table. Such behaviour questions the existence of the exotic states that hydrogen has been theoretically predicted to possess and at the very least pushes the transition pressures much higher than previously thought.
“It is remarkable to think that 4 years ago, researchers were unable to compress hydrogen above 180 GPa and 200 K, and now we are probing the melt line, far surpassing these conditions. Although this study is a major breakthrough in hydrogen research, it poses more questions about its high pressure behaviour and may even cast doubt on the existence of predicted exotic phases. Nevertheless it is very exciting to think what may occur at higher densities and will no doubt stimulate further research.” Ross Howie, Centre for Science at Extreme Conditions