Nuclear astrophysics
The light emitted by the stars is a direct result of the nuclear reactions that naturally occur when massive clouds of gas collapse and heat, whether it be the constant shine of our Sun, or the newly observed flares of gamma-ray bursters. The abundances of the elements here on Earth are also the result of nuclear reactions that have occurred in previous generations of stars. The understanding of nucleosynthesis processes and of the energy generation in astrophysical objects is the subject of Nuclear Astrophysics.
Our research aims at understanding the processes that forge the elements in stars and the big bang. This requires challenging experiments with radioactive nuclei to study the nuclear reactions taking place in explosive events such as supernovae or merging stars, and high sensitivity experiments underground, that allow us to study reactions at the very low energies occurring in stars, such as our sun. We perform experiments all around the world at leading international accelerator facilities, such as CERN (Switzerland), LUNA (Italy), RIKEN (Japan), and NSCL-MSU (USA), often with equipment we developed here in Edinburgh.
Quiescent Stellar Burning
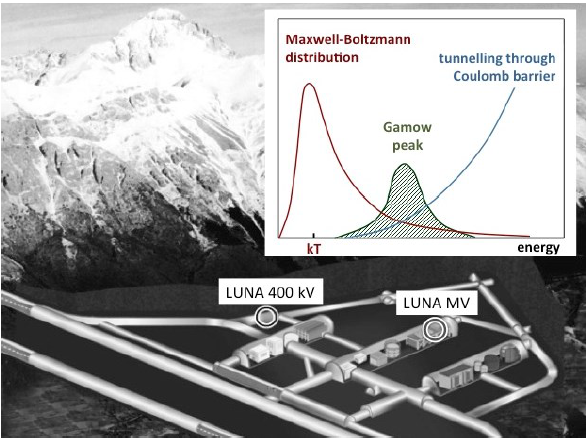
The vast majority of any star's life is spent in an almost perfect steady state of thermal equilibrium, for example stars in the main sequence such as our own Sun, or those that are in their red giant or AGB phases. Their luminosity is still powered by nuclear reactions such as those in the p-p chain or the CNO cycle occurring deep in the stellar interior, and later stages of evolution can depend on the nucleosynthesis that occurs in this quiescent period. However the lower temperatures and pressures of these stellar environments mean that the reaction rates are very small for the nuclear reactions and it is this that leads to the longevity of the stars. This can be understood in terms of the Coulomb barrier: at the temperatures involved, maybe a few millions of degrees, the corresponding kinetic energies of the particles are only a few keV, much smaller than the Coulomb barrier. This makes the direct measurement of these reaction rates in the laboratory extremely difficult.
We have an extensive experimental program at the laboratory for underground nuclear astrophysics (LUNA) which allows measurements of key quiescient burning reactions with high sensitivity at stellar energies. The new LUNA MV accelerator will become operational towards the end of 2018. This will allow for a new range of ultra-low energy reaction studies underground, including with carbon beams.
Origin of the Heavy Elements
Nearly all elements heavier than Iron are produced by neutron capture reactions. While the basic concepts of their production is understood, the origin of the heavy elements remains one of the most important outstanding problems.
About half of the heavy elements are produced in the slow neutron capture process (s-process) by a sequence of neutron captures and β-decays close to the stability valley. Current models suggest this process happens during quiescent burning phases in intermediate mass stars (1-5 solar masses) and in massive stars which later explode as core collapse supernovae. A key ingredient to determine abundances produced in this process are neutron capture crosss sections. The group has an active program of measuring important neutron induced reactions at international neutron facilities, such as n_TOF CERN and the future ultra high flux facility FRANZ (Germany).
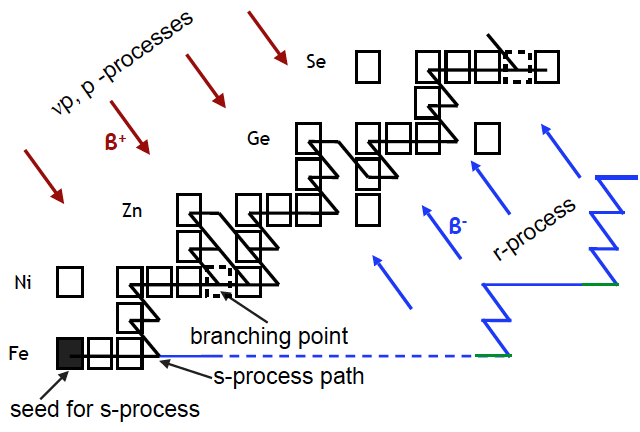
The other half of the heavy elements is thought to be produced by the rapid neutron capture process (r-process). This process happens in explosive environments of high neutron densities, and involves nuclear reactions on short-lived, exotic nuclei far from stability. At present, the astrophysical site where this process takes place remains a mystery. Recently, largely pristine r-process abundances have been observed in ultra-metal poor stars. These are providing an exciting challenge to our understanding of possible r-process sites, and require extensive new data on nuclei near, or in the region of, the astrophysical r-process. We study properties of r-process nuclei at the RIBF facility (RIKEN), using the advanced silicon strip detection system AIDA which was developed by us and collaborators. We are also founder members of the BRIKEN collaboration, where AIDA is combined with neutron detectors to measure beta-delayed neutron emission probabilities, which may alter the nucleosynthesis path in the late stages of the r-process and thus has high impact on final r-process abundances.
The abundances of a comparatively small number of rare proton-rich heavy isotopes cannot be explained by the s- or r-processes: these are the p-nuclei. They are thought to be produced by photodisintegration reactions of pre-existing seed nuclei in the outer shells of exploding massive stars. Although this process occurs naturally in supernovae, and is able to produce the bulk of p-nuclides within a single site, a longstanding problem relates to abundances of p-nuclei with A < 110 such as 92,94Mo and 96Ru which are two orders of magnitude more abundant in the solar system than expected. We explore alternative routes of their production. The vp-process may occur in core collapse supernovae, where intense neutrino winds create proton-rich ejecta with free neutrons, allowing the formation of proton-rich, heavy nuclei. We plan experiments at the new HIE-ISOLDE facility where key reactions can be studied for the first time directly at stellar energies.
Edinburgh is also leading efforts for proton capture measurements relevant for the production of p-nuclei using ion storage rings, an innovative new approach, which has recently been supported by STFC (ISOL-SRS project ST/M001652/1).
Cosmic Gamma Ray Emitters
Cosmic gamma ray emitters are long lived radionuclides that can be identified in our galaxy by their characteristic gamma ray emission when they decay. They are invaluable to study nucleosynthesis processes in stars, since detecting their presence and spatial distribution in our galaxy may help in understanding astrophysical phenomena, such as core collapse supernovae. Our research focusses on the origin of 26Al with a lifetime of about a million years. 26Al has been observed by satellite missions in our galaxy and we study key reactions that produce and destroy 26Al using a variety of experimental techniques and facilities (for example at ANL, and NSCL-MSU) which allows us to more reliably determine abundances produced in stars and supernova explosions. Another isotope of particular interest is 44Ti (half life: 60 years), which is produced in supernova explosions, and has been observed in SN1987A in much larger quantities than predicted. We are addressing this open question by studying key destruction reactions of 44Ti at ISOLDE.
PhD project opportunities in Nuclear astrophysics
- From radioactive waste to core collapse supernovae
- I Astrophysical origins of the heavy elements
- Low energy reactions for quiescent stellar evolution
- Mass measurements of the most exotic isotopes at the FRS Ion Catcher, GSI/FAIR
- Neutron desctruction of 40K
- Nucleosynthesis Calculations
- Nucleosynthesis in novae and X-ray bursts
- Origin of nature’s rarest stable isotope
- Production of proton-rich heavy elements in supernovae
- Underground measurements of key nuclear astrophysics reactions
People in Nuclear astrophysics
Telephone numbers in the list below are shown as UK numbers. Callers from outside the UK should remove the leading zero and use the UK country code (+44).
Name | Position | Contact details | Location | Photo |
---|---|---|---|---|
Academic staff | ||||
Marialuisa Aliotta | Professor of Experimental Nuclear Astrophysics | m.aliotta [at] ed.ac.uk | JCMB 8204 | ![]() |
Carlo Bruno | Chancellor's Fellow | carlo.bruno [at] ed.ac.uk | JCMB 8211 | |
Claudia Lederer-Woods | Reader | claudia.lederer-woods [at] ed.ac.uk | JCMB 8207 | |
Alex Murphy | Professor of Nuclear & Particle Astrophysics | a.s.murphy [at] ed.ac.uk | JCMB 8208 | ![]() |
Moritz Pascal Reiter | Reader | mreiter [at] ed.ac.uk | JCMB 8209 | ![]() |
Phil Woods | Professor | philip.j.woods [at] ed.ac.uk | JCMB 8203 | |
Research staff | ||||
Sid El Moctar Ahmed Maouloud | Boulby Mine PDRA | sahmedm [at] ed.ac.uk | JCMB | |
Tom Davinson | Personal Chair of Experimental Nuclear Physics | t.davinson [at] ed.ac.uk | JCMB 8205 | ![]() |
Jordan Marsh | Postdoctoral Research Associate | jmarsh2 [at] ed.ac.uk | JCMB 8206 | |
Ragandeep Singh Sidhu | Postdoctoral Research Associate | ragan.sidhu [at] ed.ac.uk | JCMB 8210 | ![]() |
Research postgraduates | ||||
Lucia Barbieri | Postgraduate Student | lucia.barbieri [at] ed.ac.uk | JCMB | |
Emmanuel Seyi Odusina | Postgraduate Student | s2562717 [at] ed.ac.uk | JCMB 8206 | |
Duncan Robb | Postgraduate Student | Duncan.Robb [at] ed.ac.uk | JCMB 8206 | |
Annie Rooney | Postgraduate Student | Annie.Rooney [at] ed.ac.uk | JCMB 8206 | |
Ashley Tattersall | Postgraduate Student | ashley.tattersall [at] ed.ac.uk | JCMB 8206 | ![]() |
Wan Aishah Wan Harun | Postgraduate Student | s2549848 [at] ed.ac.uk | JCMB |