Congratulations to Professor Peacock who has received the Royal Astronomical Society’s highest honour for his contributions to cosmology.
The Royal Astronomical Society has announced the 2023 winners of its awards, medals and prizes in recognition of significant achievement, from research to education and outreach, in the fields of astronomy and geophysics.
The Society's highest honour, its Gold Medal in Astronomy, is awarded this year to Professor John Peacock of the University of Edinburgh, in recognition of his outstanding contributions to cosmology. His work ranges from studying the early abundance of galaxies with actively fuelled central supermassive black holes, to the formation of cosmological large-scale structure and its relation to the clustering of galaxies.
Many of Professor Peacock’s contributions have been made by combining insightful theoretical analysis with the results from observational programmes. The 2dF Galaxy Redshift Survey, which he co-led, found the first convincing evidence for the influence of pressure on fluctuations in the density of ‘normal’ matter in the universe, arising in the early cosmos after the Big Bang. This work was recognised by the award of the 2014 Shaw Prize.
Alongside his major contributions to cosmology, Professor Peacock has mentored generations of students and postdocs, and written a cosmology textbook which shaped the field.
Past winners of the Gold Medal in Astronomy include Albert Einstein, Edwin Hubble, Arthur Eddington and Stephen Hawking.
The Royal Astronomical Society was founded in 1820 and encourages and promotes the study of astronomy, solar-system science, geophysics and closely related branches of science.
The MSc in Astrobiology and Planetary Sciences is the UK’s first Masters in astrobiology. The programme builds on Edinburgh’s substantial and leading role in astrobiology, geobiology and planetary sciences, including cometary studies and exoplanet science.
Astrobiology and Planetary Sciences
Astrobiology and the planetary sciences are interdisciplinary subjects that build on physics, chemistry, biology and geosciences to answer fundamental questions. Astrobiologists seek to understand life in an astronomical context: how it forms, varies and evolves in concert with planets and stars, and how it is distributed across time and space. Planetary scientists investigate the origin, diversity and behaviour of planets, moons, rings, asteroids, comets, and solar systems. Together, these sciences offer a distinctive and mind-expanding perspective on our own place in the universe.
The MSc programme will provide graduates with an exciting foundation in the rapidly advancing interdisciplinary science of life in the universe while offering methodological training for further technical or academic work in the planetary, life, or space sciences.
Key programme features
The key features of the programme are:
- Full time programme, 12 months
- Taught components in astrobiology (theory and methods), planetary sciences, and a range of optional courses from environmental geochemistry to space law
- A research dissertation
Dr Sean McMahon, MSc Programme Director said:
It’s an incredibly exciting time to be working in these fields and we very much look forward to welcoming the next generation of astrobiologists and planetary scientists to Edinburgh to participate in our programme.
Image credit: Getty images 930523318 [gorodenkoff]
Dr Cheryl Patrick has been elected as co-spokesperson for an international collaboration working to uncover the mysteries of neutrinos.
The SuperNEMO (Neutrino Ettore Majorana Observatory) collaborators are looking for evidence of neutrinoless double beta decay – a rare type of radioactive decay which has been predicted, but has never been observed.
The collaboration brings together around 100 physicists and engineers across three continents, including UK collaborators from University College London, Warwick, Manchester and Imperial College.
The collaboration uses a detector located in a tunnel at the Modane Underground Laboratory, beneath the French Alps, to search for neutrinoless double-beta decay. If this hypothesised process exists, it could give us clues to how we live in a universe of matter rather than antimatter, as well as help us to understand neutrinos - some of the most abundant, but least understood, subatomic particles in the universe.
Cheryl joined the University of Edinburgh last year as an STFC Ernest Rutherford Fellow with a research plan to uncover the mysteries of neutrinos, and SuperNEMO was at its heart. Cheryl will serve as spokesperson for the collaboration alongside Christine Marquet who is based in the University of Bordeaux’s Laboratoire de Physique des Deux Infinis Bordeaux (LP2I) group.
Cheryl, who is based in the School’s Particle Physics Experiment research group, explains:
SuperNEMO is a fantastic experiment, which uses a unique technology to help us understand double-beta decay in new and original ways. I was delighted to be able to bring Edinburgh on board, and have been lucky to find such an amazing team of undergraduate, masters and PhD students here, who have been making great contributions to the experiment. This is a really exciting time for us as we start collecting our first physics data. I can't wait to find out what we can learn about neutrinos and the double-beta decay process, and I'm honoured and humbled that the SuperNEMO family has chosen me to help lead the collaboration through such a thrilling new phase.
Congratulations to astrophysicist Dr Alex Hall who has been awarded a Royal Society University Research Fellowship.
The Royal Society’s University Research Fellowship scheme supports scientists who are in the early stages of their research path to build their career and pursue cutting-edge scientific research.
Dr Hall is based at the Institute for Astronomy. His research focuses on the fundamental physics that governs the dynamics of the Universe. Key challenges include understanding the accelerating expansion of the Universe, testing General Relativity on the largest scales, and measuring the mass of neutrinos. To answer these questions Dr Hall uses precision measurements of galaxies to infer the distribution of dark matter across the Universe. His research programme will use data from the upcoming Euclid space telescope, the European Space Agency’s flagship dark energy mission, to shed new light on some of the most important unsolved problems in physics.
Fluorescence microscopy enables measuring the mechanical properties of liquid interfaces without disturbing them.
The mechanical properties of complex liquid interfaces, i.e. interfacial rheology, are crucial for understanding the stability of emulsions and foams, including everyday examples such as skin cream and whipped cream.
Conventional techniques to measure interfacial rheology rely on macroscopic probes, like a needle or a ring, directly attached to the water-oil interface. However, this couples the interfacial flow to the bulk flows in the water and oil phases, thereby limiting measurement sensitivity. Moreover, the presence of a macroscopic probe in the water-oil interface could significantly affect the structure of the interface itself, which would mean that the mechanical properties of the measured interface are not quite the same as those of the interface without a measurement probe in it.
In their Journal of Rheology paper (and corresponding Scilight showcase), a team of researchers from The University of Edinburgh, Delft University of Technology, and the University of Gothenburg present a contactless method to measure the interfacial rheology of liquid-liquid interfaces using fluorescence microscopy.
One of the authors, Dr Job Thijssen from the School of Physics and Astronomy, explains the method: "Our typical sample is a layer of oil on top of a layer of water, with micron-sized particles attached to the interface between the water and the oil layer. We then rotate the upper (oil) layer in a controlled manner and we measure the response of the particle-laden water-oil interface using a microscope. As we control the rotation of the upper (oil) layer, we can calculate the stress (force per unit area) applied at the interface. From the microscopy videos, we can extract the strain (deformation) of the interface in response to that stress. Hence, we can quantify the mechanical properties of the interface in a flow curve (plot of stress vs shear)."
Importantly, the method should only require relatively common equipment, for example a fixed-rate motor and an optical (fluorescence) microscope, so the team hopes that their paper will contribute to interfacial-rheology measurements becoming more accessible. Through the Edinburgh Complex Fluids Partnership, they are also hoping to apply the novel contactless interfacial rheology technique to everyday and industrial samples.
Image gallery
Scientists uncover the surprising hydrogen content variability in novel lanthanum hydrides.
Superconductors
It has been a long-standing goal of physicists, chemists and material scientists to find a superconductor which works at room temperature. Such a discovery would transform our societies by allowing us to achieve more work while using less natural resources and creating reduced waste.
There are many applications which currently use materials with superconductors, such as MRI machines, radio and television broadcasting and wireless communications, electric motors and generators. At present, the highest temperature superconductors can only work under extremely high pressures, such as those near the centre of the Earth.
Lanthanum hydrides
Recent research suggests that hydrogen-based solids are among the top contenders for superconductors. Since 2015, high-pressure sciences have been at the leading edge of the synthesis of materials with high superconducting critical temperature (Tc), namely with a breakthrough Tc of 260 K reported in the lanthanum hydride (La-H) system at 1,600,000 times atmospheric pressure. Yet, incongruities in experimental measurements suggested other phases to be formed along with LaH10+δ. These undiscovered lanthanum hydrides, which also anticipated to be very high temperature superconductors, were expected to play an active role in the interpretation of the data to assess superconductivity.
Hydrogen content variability
Dr Dominique Laniel led a team of international collaborators from the University of Bayreuth and Linköping University in employing extreme pressure and temperature conditions to synthesize seven lanthanum hydrides, including five of which were previously unobserved.
A detailed investigation of these hydrides determined that their hydrogen content for a given lanthanum atoms’ arrangement varies depending on pressure and their synthesis conditions—an observation key to understanding pressure-formed hydrogen-based superconductors.
The team synthesized five novel lanthanum hydrides: LaH~4, LaH4+δ, La4H23, LaH6+δ and LaH9+δ, along with the previously known LaH3 and LaH10+δ. To accomplish this, lanthanum and paraffin—i.e. a hydrogen reservoir—were first squeezed to enormous pressures of 0.96 to 1.76 million times the atmospheric pressure in between two tiny diamond anvils and heated to temperatures of more than 2200°C using high power lasers. Then, to characterise the atomic arrangement adopted by the compounds under these conditions, the samples were illuminated by an intense X-ray beam at two particle accelerators, the German PETRA III and the United States APS synchrotrons.
Dr Laniel commented:
Given the extensive investigations that the La-H system had already underwent, we were surprised to see the formation of this many previously unknown lanthanum hydrides. But the most unexpected result was that their hydrogen-content seemed to greatly vary based on their synthesis condition and pressure—without any noticeable changes in the lanthanum atoms’ arrangement.
This discovery, which is published in Nature Communications, has profound ramifications. Largely due to hydrogen having a single electron, this element cannot directly be detected by X-ray diffraction: a method commonly used to study these hydrides. As such, scientists instead rely on theoretical calculations to determine the position and content of hydrogen atoms in these solids. However, such calculations often assume a single possible configuration of hydrogen atoms for a given arrangement of lanthanum atoms—a hypothesis now demonstrated to be incorrect. This realisation is especially crucial given the very strong dependence of a solid’s superconducting temperature on the hydrogen atoms, i.e. having the wrong model for the hydrogen atoms’ is likely to completely change the calculated superconducting temperature, in turn greatly undermining the reliability of theoretical calculations.
Upon re-analysing published data from other groups, Dr Laniel and collaborators found this hydrogen content variability to be a recurrent phenomenon, thereby generalizing their observations to other systems.
This established hydrogen content variability will undoubtedly provide the impetus for revising theoretical models of high pressure hydrides, ultimately leading to an intimate understanding of their superconductivity and bringing us one step closer to a room temperature superconductor.
Image gallery
Investment received to support the UK’s academic and industrial strengths in biofilm research and innovation.
The Biotechnology and Biological Sciences Research Council (BBSRC) and Innovate UK will invest £7.5m to support the National Biofilms Innovation Centre (NBIC), expanding on its world-class research and innovation.
The University of Edinburgh is one of NBIC’s four lead research institutions, conducting important research into microbial biofilms across several schools, institutes and research centres, including input from a team at the School of Physics and Astronomy. This research spans a wide range of topics and areas such as bioengineering, biofilm formation, inflammation research, infection control, hygiene and wastewater treatment. The study of biofilms spans disciplines, from understanding how single cells become complex multicellular systems, to collaborative research on how to eradicate biofilms or prevent bacterial infections.
National collaboration
Launched in 2017 by its four lead universities: Edinburgh, Liverpool, Nottingham and Southampton, this UK Innovation and Knowledge Centre has expanded partnerships with 59 research institutions and more than 150 companies across the UK.
NBIC has secured a further £9.5m from the lead universities as well as £6.4m industrial support, taking its Phase 2 funding to a total of £23.4m.
Tackling global challenges
The latest funding will support NBIC’s vision to deliver a global innovation hub by building on its collective strengths to prevent, detect, manage and engineer biofilms. It will enable NBIC to drive the adoption of innovative solutions across industry sectors to address major global challenges including climate change, water safety and improved healthcare. It will also drive step-changes in standards and regulation for novel biofilm solutions that support international trade. Phase 2 will also see NBIC deliver a diverse training programme to equip the biofilm innovation ecosystem with the skills they need both now and, in the future, while also nurturing the talent of tomorrow.
What are biofilms?
Biofilms are central to our most important global challenges, from antimicrobial resistance and food safety to water security. They also provide a significant contribution to both the UK and global economy. In May 2022, a study carried out by NBIC estimated that the value of the markets in which biofilms are involved is worth £45 billion in the UK and $4 trillion globally.
NBIC University of Edinburgh Co-Director, Professor Cait MacPhee, who is based in the School of Physics and Astronomy said:
I am delighted that the pioneering work of NBIC will continue with Edinburgh as one of the core partners. Microbial biofilms play a key role in the biosphere, and are important to environmental efforts such as remediation and the safe maintenance of large-scale infrastructure. They also have an impact, both positive and negative, on a variety of different industries across multiple sectors, including healthcare. We will continue with our work to link the best academic research across the UK to meet urgent industrial needs, for the benefit of end-users and the general public. Anyone with an interest in biofilms is encouraged to get in touch.
Image gallery
Edinburgh astronomers awarded UK Space Agency funding for international mission.
A consortium of UK astronomers, led by Professor Andy Taylor at the School’s Institute for Astronomy, has been awarded £8 million by the UK Space Agency over the next two years to develop and launch an international space mission to study dark energy and dark matter in the Universe.
The Euclid satellite, expected to be launched in 2023, is a European Space Agency (ESA) flagship mission to image and measure the distance to over 1.5 billion galaxies over 15,000 square degrees of the sky, looking back over half the age of the Universe.
The high-quality images will be carefully processed to generate maps of the distribution and evolution of dark matter and galaxies across the Universe. The maps will be analysed to determine the nature of dark energy, the mysterious phenomena which is driving the accelerated expansion of the Universe.
The UK Space Agency funding will support UK astronomers and computing specialists who are developing advanced methods for the Euclid Science Ground Segment data analysis, and the UK’s dedicated Euclid Science Data Centre, hosted at Edinburgh. The UK consortium is a major component of the Euclid Consortium, a wider European-led network of scientists working on the Euclid mission.
Image gallery
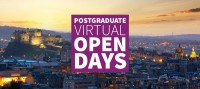
The School of Physics & Astronomy will take part in the University of Edinburgh Postgraduate Virtual Open Days.
The School of Physics and Astronomy events will consist of presentations and Q&A sessions on the following days:
- Wednesday 16th November: Introduction to MSc Theoretical Physics and Mathematical Physics 14:00-15:00 UK time
- Wednesday 16th November: Introduction to MSc Particle and Nuclear Physics 16:00-17:00 UK time
- Thursday 17th November: Q&A with MSc students and alumni 14:30-15:30 UK time
To book a place visit the Virtual Postgraduate Open Days 2022 page on the University of Edinburgh website.
Congratulations to Dr Carlo Bruno who has been awarded the Young Investigator prize in Nuclear Physics.
The Young Investigator prize in Nuclear Physics is awarded to recognize and encourage promising experimental or theoretical research in nuclear physics, including the advancement of a method, a procedure, a technique, or a device that contributes in a significant way to nuclear physics research.
Carlo Bruno was awarded this prize for his experimental work with low-energy nuclear reactions relevant for astrophysics and his leading role in transferring these experiments into storage rings using radioactive beams.
Carlo graduated from the Università Statale di Milano in Italy, and came to the School’s Institute for Particle and Nuclear Physics for his PhD in Nuclear Astrophysics, which he obtained in 2017. Carlo's research focuses on measuring nuclear reactions of key importance to understand the origin of the elements from the Big Bang to supernovae using heavy ion storage rings at FAIR (Germany). He has played a leading role in creating CARME (CRYRING Array for Reaction Measurements), an extreme vacuum chamber for use in international nuclear and atomic physics experiments.
This prize was established by the International Union of Pure and Applied Physics (IUPAP), which works to assist in the worldwide development of physics, to foster international cooperation in physics, and to help in the application of physics toward solving problems of concern to humanity.